Origin of the Elements

Approximately 73% of the mass of the visible universe is in the form of hydrogen. Helium makes up about 25% of the mass, and everything else represents only 2%. While the abundance of these more massive ("heavy", A > 4) elements seems quite low, it is important to remember that most of the atoms in our bodies and Earth are a part of this small portion of the matter of the universe. The low-mass elements, hydrogen and helium, were produced in the hot, dense conditions of the birth of the universe itself. The birth, life, and death of a star is described in terms of nuclear reactions. The chemical elements that make up the matter we observe throughout the universe were created in these reactions.
Approximately 15 billion years ago the universe began as an extremely hot and dense region of radiant energy, the Big Bang. Immediately after its formation, it began to expand and cool. The radiant energy produced quark-antiquarks and electron-positrons, and other particle-antiparticle pairs. However, as the particles and antiparticles collided in the high energy gas, they would annihilate back into electromagnetic energy. As the universe expanded the average energy of the radiation became smaller. Particle creation and annihilation continued until the temperature cooled enough that pair creation became no longer energetically possible.
One of the signatures of the Big Bang that persists today is the long-wavelength radiation that fills the universe. This is radiation left over from the original explosion. The present temperature of this "background" radiation is 2.7 K. (The temperature, T, of a gas or plasma and average particle kinetic energy, E, are related by the Boltzmann constant, k = 1.38 x 10-23 J/K, in the equation E = kT.) Figure 10.1 shows the temperature at various stages in the time evolution of the universe from the quark-gluon plasma to the present time.
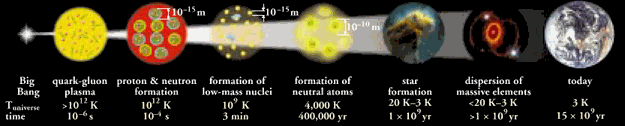
At first quarks and electrons had only a fleeting existence as a plasma because the annihilation removed them as fast as they were created. As the universe cooled, the quarks condensed into nucleons. This process was similar to the way steam condenses to liquid droplets as water vapor cools. Further expansion and cooling allowed the neutrons and some of the protons to fuse to helium nuclei. The 73% hydrogen and 25% helium abundances that exists throughout the universe today comes from that condensation period during the first three minutes. The 2% of nuclei more massive than helium present in the universe today were created later in stars.
The nuclear reactions that formed 4He from neutrons and protons were radiative capture reactions. Free neutrons and protons fused to deuterium (d or 2H) with the excess energy emitted as a 2.2 MeV gamma ray,
n + p Æ d + g.
These deuterons could then capture another neutron or free proton to form tritium (3H) or 3He,
d + n Æ 3H + g and d + p Æ 3He + g.
Finally, 4He was produced by the reactions:
d+ d Æ 4He + g, 3He + n Æ 4He + g and 3H + p Æ 4He + g.
Substantial quantities of nuclei more massive than 4He were not made in the Big Bang because the densities and energies of the particles were not great enough to initiate further nuclear reactions.
It took hundreds of thousands of years of further cooling until the average energies of nuclei and electrons were low enough to form stable hydrogen and helium atoms. After about a billion years, clouds of cold atomic hydrogen and helium gas began to be drawn together under the influence of their mutual gravitational forces. The clouds warmed as they contracted to higher densities. When the temperature of the hydrogen gas reached a few million kelvin, nuclear reactions began in the cores of these protostars. Now more massive elements began to be formed in the cores of the very massive stars.
Chapter Contents
|