BERKELEY, CA — For the first
time, researchers have made direct images of the alignment of magnetic
domains on both sides of an interface between ferromagnetic and
antiferromagnetic films -- an example of "pinning" in the kind
of layered magnetic structure vital to today's advanced computer recording
heads and to the memory devices of the future. Their accomplishment is
reported in the June 15, 2000, issue of the journal Nature.
 |
CIRCULARLY POLARIZED X RAYS IMAGE THE MAGNETIC DOMAINS IN THE
FERROMAGNETIC LAYER, WHICH IS IDENTIFIED BY THE EMISSION OF
ELECTRONS FROM COBALT. LINEARLY POLARIZED X RAYS IMAGE
MAGNETIC DOMAINS IN THE ANTIFERROMAGNETIC LAYER, IDENTIFIED BY THE
EMISSION OF ELECTRONS FROM IRON. AT THE INTERFACE OF THE TWO
KINDS OF MATERIALS, THE DOMAINS ARE PRECISELY ALIGNED.
|
At the Advanced Light Source (ALS), located at the Department of
Energy's Lawrence Berkeley National Laboratory, a consortium of
researchers from Berkeley Lab, Stanford University, IBM Corporation, the
University of Neuchâtel in Switzerland, and Arizona State University
obtained images of tiny magnetic domains in cobalt, a ferromagnet, coupled
to domains with the same orientation in an adjacent layer of lanthanum
iron oxide, an antiferromagnet. The phenomenon is known as exchange bias,
or pinning.
"The exchange bias phenomenon has been known for more than 45
years, but even though we have used the effect and even built
sophisticated devices by trial and error, we haven't understood how it
works," says Frithjof Nolting, an ALS researcher visiting from
Stanford University.
In ferromagnets, the electronic spins in magnetic domains are aligned
parallel to one another. In antiferromagnets they alternately point in
opposite directions, which renders antiferromagnets insensitive to applied
magnetic fields.
When these materials are layered to create computer read-heads or other
magnetization sensors, one ferromagnetic layer is pinned by an adjacent
antiferromagnetic layer -- and thus acts as a magnetic reference -- while
another is free to change its orientation in response to an applied field.
When the spins of the ferromagnetic layers are opposed, resistance to
an electric current is greater than if they are parallel; this is the
so-called "giant magnetoresistance" effect. By varying the
orientation of domains in one of the ferromagnetic layers and thus varying
the resistance, information can be written, stored, and recovered.
For a better understanding of pinning, Nolting says, two things were
needed: "first, a method of imaging the configuration of domains in
antiferromagnetic thin films, which requires a resolution better than 100
nanometers" -- 100 billionths of a meter -- "and second, a way
to image the interface between ferromagnetic and antiferromagnetic domains
in adjacent layers," which requires distinguishing between layers
containing different chemical elements.
PEEM2, the photoemission electron microscope at ALS beamline 7.3.1.1,
meets these requirements. When an x-ray beam is incident upon a sample,
electrons ejected from the sample can be used to form an image with
ten-thousand-fold magnification and a resolution of 20 nanometers.
X rays of different energies stimulate photoelectrons characteristic of
different elements; for example, near 710 eV (710 electron volts) iron
emits copious photoelectrons, while cobalt does the same near 780 eV. By
tuning the energy of the beam, layers containing different elements can be
distinguished.
If the x-ray beam is polarized, it can reveal magnetic domains: linear
polarization produces high-contrast images of antiferromagnetic domains;
circular polarization yields high-contrast images of ferromagnetic
domains.
In the sample used by the researchers, a cobalt film less than three
nanometers thick was deposited on a film of lanthanum iron oxide. "By
tuning the photon energy of the beam, we were able to record separate
images of the antiferromagnetic and ferromagnetic layers in exactly the
same place," Nolting says.
The resulting perfectly registered images show precise correspondence
between the spin orientation of microscopic domains in the lanthanum iron
oxide layer and the domains of the ferromagnetic layer immediately
adjacent to them, demonstrating that magnetic exchange coupling aligns the
magnetic structure of both layers, domain by domain.
When they measured the strength of the coupling by applying a magnetic
field, the researchers discovered an unexpected phenomenon.
Because exchange-bias devices such as read heads depend upon an overall
preferred magnetic orientation in a ferromagnetic layer, which is achieved
by its coupling to the antiferromagnet, a "bias" is set during
the manufacturing process.
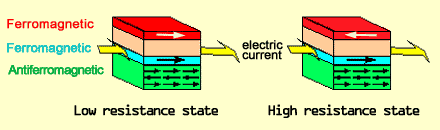
REFERENCE FERROMAGNETIC LAYER (BLUE) IS PINNED BY EXCHANGE BIASING TO
ANTIFERROMAGNETIC LAYER (GREEN)
"The usual method is to set a bias by annealing the multilayer in a
magnetic field," Nolting explains. "But we imaged samples just
as they were grown, without any additional processing. And we found that
prior to any setting procedure, there is already a bias locally, within
each individual domain" -- although because of their random
orientation, the effect vanishes over large areas.
"Apparently exchange bias is an intrinsic property of the
interface, caused by the common alignment of the magnetic structure of
both materials," Nolting adds, "even though initially there may
be no total bias, averaged over a large area."
By using photoemission electron microscopy at the ALS, the researchers
were able to make the first direct observation of the link between
antiferromagnetic and ferromagnetic spin at the interface between the two
kinds of materials. Their measurements imply that the setting procedure
reorients domains, shifting the balance from a random bias in opposing
directions to a preferred bias direction. But it does not create that
bias.
"This opens the door to new investigations, which may affect the
way devices based on the exchange bias effect are manufactured and the
materials that can be used in them," says Nolting.
Frithjof Nolting and his colleagues report their findings in a letter
to Nature, "Direct observation of the alignment of
ferromagnetic spins by antiferromagnetic spins," June 15, 2000.
Other authors of the letter are Andreas Scholl of the ALS; Joachim
Stöhr of Stanford University, formerly of the IBM Almaden Research Center
in San Jose, who led the project; Jin Won Seo of the University of
Neuchâtel and IBM's Zürich Research Laboratory; Jean Fompeyrine, Heinz
Siegwart, and Jean-Pierre Locquet of IBM's Zürich Research Laboratory;
Simone Anders of the ALS; Jan Lüning (now with the Stanford Synchrotron
Radiation Laboratory), Eric. E. Fullerton, and Michael F. Toney of IBM's
Almaden Research Center; Michael R. Scheinfeld of Arizona State
University; and Howard A. Padmore of the ALS.
The Berkeley Lab is a U.S. Department of Energy national laboratory
located in Berkeley, California. It conducts unclassified scientific
research and is managed by the University of California.
Additional Information:
|