|
 |
Less than a year ago the National Cancer Institute launched a multidisciplinary
"program project" named Structural Cell Biology of DNA Repair
Machines (SBDR), aimed at elevating the understanding of DNA repair from
the level of individual protein structures to the level of complex interactions
within the cell.
On August 1, Nature published the project's first major result,
the unexpected discovery of the Rad50 "zinc hook," a metal-mediated
interface that the essential Mre11/Rad50 protein complex uses to link
broken DNA strands so that they can be rejoined.
|
 |
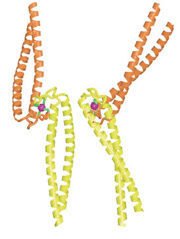 |
|
|
 |
The coiled-coil tails of Rad50 proteins
link to each other with zinc hooks. |
|
|
SBDR is a $18 million program involving 18 investigators at 11 institutions,
pursuing five research programs in three major areas of DNA repair. Based
at Berkeley Lab, SBDR's principal investigator is John Tainer, a professor
of molecular biology at the Scripps Research Institute, with a visiting
faculty appointment in Berkeley Lab's Life Sciences Division. Co-principal
investigator is Priscilla Cooper, acting director of the Life Sciences
Division.
"We believe that SBDR will be powerful for providing insights on
the function of dynamically assembled DNA repair machines," says
Tainer. "These insights absolutely require advanced technologies
that bridge the gaps from molecular structure to assemblies to repair
networks to cell biology. Such a combination of technologies cannot be
found in individual laboratories but instead requires a highly collaborative
effort."
Cooper adds, "The huge advantages we get from basing the SBDR Program
at LBNL come directly from the broad base of biological expertise in DNA
damage and repair in the Life Sciences Division, coupled to the structural
expertise and advanced biophysical technologies at the Advanced Light
Source. The established strength of Berkeley Lab in mounting and sustaining
large multidisciplinary programs provides an added major advantage."
Understanding complex molecular machines requires an arsenal of techniques,
among them traditional molecular biology and biochemistry laboratory methods,
electron microscopy, mass spectrometry, and the use of synchrotron radiation.
An essential component of SBDR will be the versatile SIBYLS beamline
funded by the Department of Energy and now being constructed by the same
team who built the Advanced Light Source's highly successful superbend
beamlines for structural biology, led by Dave Plate and Nicholas Kelez
under the guidance of physicist Alastair MacDowell and ALS Experimental
Systems Group leader Howard Padmore.
SIBYLS, an acronym for "structurally integrated biology for life
sciences," will have the unique capability of performing both x-ray
crystallography, to determine the structure of crystallized proteins,
and small-angle x-ray scattering (SAXS), which studies proteins in solution,
conditions more nearly resembling their natural environment. When the
crystal structures of individual proteins are known, SAXS can sometimes
be used to reveal how they work together in complexes; it can also be
an important tool for gaining structural information on impossible-to-crystallize
proteins.
Life under siege
DNA is incessantly attacked outside and inside the organism by radiation,
toxic chemicals, and other factors, and can also be damaged by accidents
of cell division. Some of the results are chemically corrupt single bases,
groups of missing or altered bases, the wrong base pairs in a gene sequence,
and broken single or double strands of DNA. Because damaged DNA contributes
to birth defects, developmental abnormalities, cancers, and other diseases,
constant repair of DNA is one of the most basic functions of life.
Different proteins act together along different pathways to repair different
kinds of damage; some groups have been doing DNA repair for millions or
billions of years and are found in organisms from bacteria to humans.
While the specific proteins involved in a particular repair process are
not always identical among different organisms, their group geometries
and functional "strategies" are often similar.
|
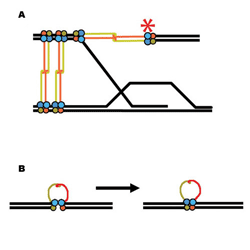 |
 |
|
|
Two of the many ways the Mre11/Rad50
protein complex could help repair damaged DNA: (A) recombination with
a sister chromatid; (B) bringing together broken double strands |
|
|
|
"Previously DNA repair was thought of as a collection of clean,
neat, separate pathways, but now we know that these pathways are interwoven,"
says Susan Tsutakawa of the Life Sciences Division, a crystallographer
in SBDR's molecular biology lab. "Proteins that were thought to have
a role in only one pathway are now understood to be involved in many."
The proteins Mre11 and Rad50, for example, form repair complexes found
in all kingdoms of life; the Mre11 complex's remarkably diverse functions
include roles in cell division, maintenance of chromosome-capping telomeres,
and repairing double-stranded breaks in DNA in two different ways. The
August 1 Nature paper by Tainer and his colleagues compares the Mre11
complex in eight organisms: a virus, an archaeon, a bacterium, two yeasts,
a nematode, a plant, and humans. They found a new and surprising mechanism
by which the Mre11 complex accomplishes DNA repair.
DNA repair, part 2
|