Goals & Challenges (page 2)
CHALLENGE: Develop efficient catalysts molecules to drive the energy storing chemical reaction
While PV elements of various designs are essential building blocks in photoelectrochemical cells, probably the greatest scientific challenge is the development of viable fuel-forming and oxygen-evolving catalysts.
Catalysts for three types of chemical transformations are particularly relevant, namely the reduction of carbon dioxide to methanol, proton reduction to hydrogen, and oxidation of water to oxygen (for the fuel generation and use cycle to be closed, water is the only viable electron source for fuel formation). The catalysts are required to handle multiple electron transfer steps, and a particular challenge is to invent systems made of abundant, non-precious metals. Catalysts need to run at rates sufficiently high for the fuel formation and water oxidation to keep up with the solar flux so that no solar photons are wasted. Also, they need to work at low overpotential, which assures that a maximum fraction of the solar light energy is converted to chemical energy of the fuel.
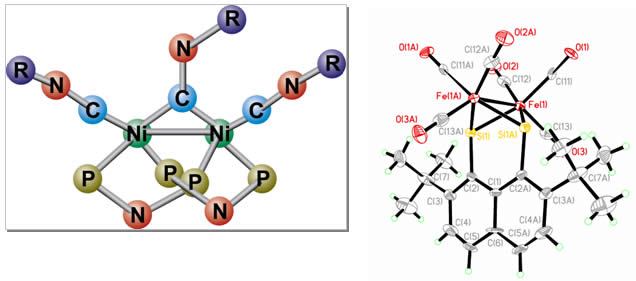
Above is a catalyst that reduces carbon dioxide (CO2) to carbon monoxide (CO) and water molecules. The active core of the catalyst consists of two linked Ni atoms. When the Ni core receives electrons from a cathode or a charge separating molecule, it activates CO2 which then splits into CO and H2O.
The structure on the right is a catalyst capable of reducing protons to hydrogen molecules. By manipulating the structure and electronic properties of the organic ligands, the properties of the Fe dimer core are fine-tuned for efficient activation of protons and conversion to H2.
The development of efficient electrocatalytic systems requires new analytical methods to observe and manipulate chemical processes with high spatial and temporal resolution on the electrode surface. In this way, the most active centers on the electrode can be identified and new electrodes designed that operate with improved efficiency. Effort of the research teams is on the development of novel instrumentation to enable such studies, and of computational and theoretical tools for describing reaction intermediates and ultimately the full sequence of chemical and charge transport processes on electrodes.
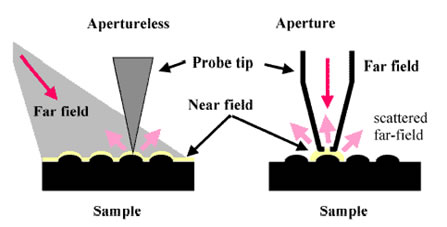
Imaging and spectroscopy are used to study catalytic sites on electrode surfaces on the length scale of nanometers. These studies rely on new instruments with high spectral and temporal resolution and the latest advances in atomic microscopy and near field Raman techniques.
The durability of the components is a major aspect of the viability of an artificial photosystem, which is the reason for our emphasis on structures made of robust inorganic materials. Nevertheless, soft structures may be the best materials choice for some components because of the ease of fine tuning electronic properties of organic matter. However, protection against photooxidative damage is essential for enhancing the durability of such components, particularly under excessive exposure to sunlight. Nature utilizes elaborate light regulation and protection mechanisms to assure the durability of its protein, lipid and other organic structures. Inspired by knowledge emerging from biological systems, SERC researchers are developing light protection for soft matter parts of engineered solar fuel generators.
CHALLENGE: Integrate the components into a viable artificial photosynthetic system
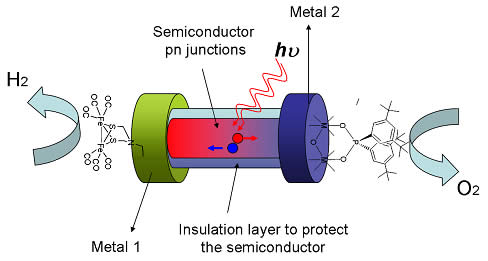
A molecular catalyst for water oxidation is covalently attached to the cathodic side of a nano PV element. When the nano PV absorbs sunlight, positive and negative charges are driven to opposite ends of the nano PV. Upon capture of positive charges, the catalyst activates water molecules, and O2 is produced. A proton-reducing catalyst attached with a robust chemical link to the opposite, anodic end of the nano PV generates hydrogen when activated by negative charges.
Putting the light absorbing, charge separating and catalytic components together into an integrated system that efficiently converts sunlight to fuel requires breakthroughs on several scientific fronts. One area is the coupling of the catalysts to PV elements in a way that allows directional transport of charges across robust linkages with minimal loss of energy. Teams are exploring synthetic methods to build efficient contacts and are employing ultrafast spectroscopy and theoretical simulations to characterize and understand the electron flow across these linkages. The goal is to design contacts that result in minimal loss of charge and energy.
A most persistent obstacle towards efficient artificial photosynthetic systems is the coupling of the water oxidation photocatalysis with the reducing, fuel generating steps. The coupling needs to be done in a way that results in evolution of oxygen and fuel molecules in physically separated spaces, in order to prevent back reaction. SERC teams are exploring new types of nanostructured membranes that allow the arrangement of photoactive and catalytic components with defined orientation using a variety of approaches that range from inorganic nanotube arrays to block copolymer based constructs.
Maximum conversion of solar light to chemical energy of a fuel demands meticulous fine tuning of the electrical potential of light absorber, catalyst, and the charge transport components that link the water oxidation and carbon dioxide reduction units. Molecular structures are particularly well-suited for manipulating electronic properties, and if they are of all-inorganic metal oxide type, they possess the required robustness for the harsh conditions of artificial photosynthesis. SERC researchers are assembling molecularly defined all-inorganic components with carefully matched potentials in a nanostructured silica scaffolds, then use the latest tools of nanoscience for creating a hierarchical photosynthetic membrane that affords instant separation of oxygen from reduced carbon dioxide products.
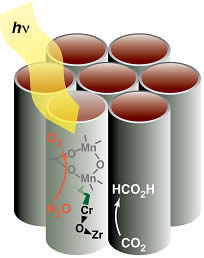
Photoactive units for light absorption, charge separation and catalysis made of abundant transition metals are assembled atom by atom in ananoporous silica scaffold (gray tubes). The oxo bridged unit consisting of a Zr and Cr atom is the light absorbing electron pump that drives a water oxidation catalyst. On the Zr end, carbon dioxide is activated. The all-inorganic photocatalytic unit is positioned such that oxygen evolves in a space separated from that of the carbon dioxide reduction products.