|
 |
A highly sensitive experiment now underway to find the axion,
a mysterious species of subatomic particle that may or may not exist, could be made even
more sensitive in the next generation of the experiment. The development of a radio
frequency amplifier based on a new type of high-gain, ultra-low noise Superconducting
QUantum Interference Device (SQUID) should make it possible for the first time ever to
detect even the most elusive axions. The new amplifier uses a direct current SQUID made
from superconducting niobium and configured so that its input coil is used as a microstrip
resonator. With this unique configuration, the input signal is coupled between one end of
the input coil and the SQUID loop which acts as a groundplane for the microstrip
resonator. The result is a radio frequency amplifier operating at frequencies approaching
1.0 gigahertz the highest at which a SQUID amplifier has ever been successfully
operated.
 |
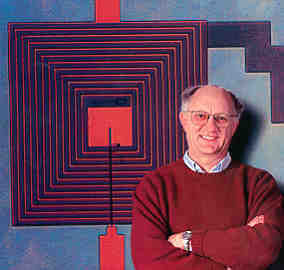
JOHN CLARKE, PICTURED WITH AN ENLARGED PHOTO OF A HIGH TC SUPERCONDUCTING COIL
DEPOSITED ON A HIGH-TC SQUID
|
This amplifier was developed by the research group of John Clarke, a physicist who
holds a joint appointment with the U.S. Department of Energy's Lawrence Berkeley National
Laboratory and the University of California at Berkeley. Key contributions were made by
group members Marc-Olivier André and Michael Mück.
One of the first applications of the new amplifier will be to enhance what is already
the most sensitive search for axions in science. An axion is a hypothetical elementary
particle that has been proposed to explain the absence of an electrical dipole moment for
the neutron. If it exists, an axion would have neither electric charge nor spin and a mass
that might be a trillion times less than that of an electron.
Despite its slight presence, the axion is widely considered to be an excellent
candidate for explaining the nature of "dark matter" in the universe. Even in
light of recent new findings about the Cosmological Constant, there still seems to be an
enormous amount of matter in the Universe which cannot be seen but which makes its massive
gravitational presence known through the motions of the galaxies. Axions could help
explain dark matter through sheer numbers; an estimated 100 trillion of them are packed
into every cubic centimeter of space in our galaxy. But first, scientists have to prove
they exist.
An experiment to detect axions began in 1995 at the Lawrence Livermore National
Laboratory (LLNL). This ongoing experiment is led by LLNL physicist Karl van Bibber and
physicist Les Rosenberg of the Massachusetts Institute of Technology. In addition to
scientists from LLNL and MIT, it also involves Clarke and his group at Berkeley Lab/UC
Berkeley, plus scientists from the University of Florida, the University of Chicago, and
Fermi National Accelerator Laboratory.
In this experiment, the static magnetic field of a powerful (8 Tesla), 12-ton
superconducting electromagnet is used to stimulate axions to decay into microwave photons
via the "Primakoff effect." An excess of microwave photons above thermal and
electronic noise signals the decay of an axion. This signal is extremely faint, however,
and must be amplified in order to be detected.
With the addition to the experiment of the Clarke group's radio frequency amplifier and
its niobium SQUID, signals from the decay of dark-matter axions should get a much needed
boost. Clarke and his group report they have obtained resonant frequencies 200 MHz to
720MHz and gains of about 20 decibels. What this would mean to the axion search, according
to van Bibber and Rosenberg, is that for the first time, the experiment should be able to
reach weakly-coupled axions at fractional halo density. These axions are considered to be
the most promising of the axion-based dark-matter models.
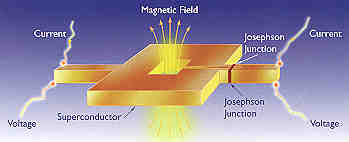
A SQUID IS THE MOST SENSITIVE TYPE OF DETECTOR KNOW TO SCIENCE.
COMPOSED OF A SUPERCONDUCTING LOOP WITH TWO JOSEPHSON JUNCTIONS, SQUIDS ARE USED TO
MEASURE MAGNETIC FIELDS. |
|