|
 |
|
 |
|
|
THE FIRST SUCCESSFUL CYCLOTRON
|
|
In the late 1920s and early 30s physicists were anxious to accelerate
subatomic particles to a million volts, the going estimate of the energy
needed to penetrate the Coulomb barrier of the nucleus. There were many
ingenious schemes to deliver ions with million-volt potentials more or
less all at once.
Although Van de Graaff generators and Cockroft-Walton machines still
have their uses, the importance of high-tension devices began to decline
after the annual meeting of the National Academy of Sciences in Berkeley
in September, 1930. Reporting from that first NAS meeting west of the
Mississippi, the New York Times announced that a "new apparatus to
hurl particles at a speed of 37,000 miles per second in an effort to obtain
a long-sought goal the breaking up of the atom was described
here today by Professor Ernest O. Lawrence of the University of California."
Lawrence, inspired by Rolf Wideröe's sketch of a linear "resonance
accelerator" in a German electronics journal but skeptical of his
own bright idea, had waited more than a year to ask a graduate student,
Niels Edlefsen, to build a working model of the cyclotron in 1930. The
result looked like a squashed perfume bottle smeared with candle wax,
and although it persuaded Lawrence, there is some doubt that it actually
worked.
By January 1931 a four-inch brass cyclotron built by another graduate
student, M. Stanley Livingston, had accelerated protons to 80,000 electron
volts with only a thousand volts on its electrodes, and Lawrence was already
raising money for a bigger magnet. By August an 11-inch cyclotron had
accelerated protons to 1.1 million electron volts.
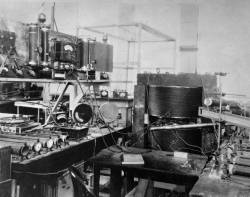 |
|
THE 11-INCH CYCLOTRON, SHOWN INSTALLED
IN ROOM 329 LE CONTE HALL, WAS THE FIRST CYCLOTRON TO EXCEED 1 MeV. |
|
In concept, a cyclotron is an ingeniously simple device. Start with a
flat round vacuum can and insert two semicircular electrodes, the "dees,"
back to back. Put this arrangement between the poles of a strong magnet.
Now introduce protons or other ions into the center of the can.
As voltage switches back and forth between the dees, the protons are
attracted first one way then the other; in the magnetic field, this acceleration
makes them move in a spiral. Each time a proton changes sides it gets
a little boost, moving faster and spiraling wider. Here's the beauty of
it: the faster it moves, the farther it travels, and the time to complete
each 360-degree circuit is always the same the protons are naturally
synchronized with the oscillating voltage. By the time they reach the
edge and exit through a window, they have acquired a lot of energy, a
little at a time.
Because cyclotrons seemed a relatively inexpensive path to high energies,
laboratories all over the world built them in the 1930s, first in other
American universities and then in Japan -- RIKEN's was the first to function
outside the U.S. ?? and in the Soviet Union, the United Kingdom, Denmark,
Germany, France, and Sweden.
If the cyclotron principle is simple, however, its practice is an art.
Historians J. L. Heilbron and Robert W. Seidel put it this way: "a
week's sweating over a Berkeley cyclotron was worth six months' immersion
in its blueprints." In the decade before World War II, the world's
leading experimental physicists beat a path to Lawrence's door.
The war brought an end to the reign of the cyclotron per se as the premier
instrument of high-energy physics. Parts of the 184-Inch Cyclotron under
construction in 1941 were diverted to defense work, and by the time it
was possible to complete the massive structure, it was looking more than
a little ungainly.
In 1945 Lawrence's longtime colleague Edwin McMillan came up with the
idea of "phase stability" (as had the Soviet scientist Vladimir
Veksler, independently), which applies to all kinds of accelerators. Together
with frequency modulation, this alternative approach to keeping bunches
of particles in sync with an accelerating field suggested a way of overcoming
the classic cyclotron's "relativistic barrier," which arises
as particles approach the speed of light, acquire mass, and fall out of
synchronization. The 184-Inch was redesigned as a "synchro-cyclotron"
and gave its first beam late in 1946.
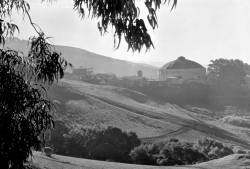 |
|
Today the world's highest-energy accelerators, from Fermilab's Tevatron
to the Large Hadron Collider under construction at CERN, owe their ring
shape to the cyclotron, a "linear accelerator wrapped in a circle."
Unlike linear accelerators, where each accelerating gap acts on the beam
only once, ring machines depend upon the repeated use of an accelerating
structure a dee in the cyclotron, a radio-frequency cavity in later
designs through which the beam circulates over and over. All accelerators,
whether rings or linacs, depend on the principle of phase stability inspired
by the 184-Inch Cyclotron and applied in its rebirth as the Synchro-Cyclotron.
Real cyclotrons are no longer used for high-energy physics. They are
nevertheless ubiquitous essential to nuclear science and other
basic research, and vital to medicine.
|