PEEM2, a second generation photoemission electron microscope,
has been operating at the Advanced Light Source for over
two years, and, remarks Andreas Scholl, leader of the
PEEM2 team, "we have shown that this instrument gets
good results" -- which is quite an understatement,
says Jun Feng of the ALS.
"PEEM2 is the most sophisticated microscope of its
type in the world," Feng says, one that has been
responsible for a string of benchmark articles in major
scientific journals. But while PEEM2 can resolve features
separated by just tens of nanometers (billionths of a
meter) -- ten times better than the best optical microscopes
-- the structures of many complex materials are even smaller.
"Characterizing these materials at ultrahigh spatial
resolution is essential to scientific and technological
progress," says Feng. His hope is to achieve resolution
ten times better yet, of one or two nanometers, "the
ultimate possible."
Feng's team is one of two in the world who are racing
to build the next generation PEEM. A collaboration of
German universities, research institutions, and corporations
called SMART has a head start, but Feng and his colleagues
are confident that the experience and resources of their
tightly-knit PEEM3 design group, centered at Berkeley
Lab, can catch up -- and in the process add some unique
features, particularly for the study of magnetic materials.
How a PEEM works
When a beam of synchrotron x rays is focused on a sample,
the material emits electrons whose energy and trajectory
convey a great deal of information. PEEMs collect and
focus these electrons with a system of magnetic and electrostatic
lenses.
The resulting image not only gives a physical picture
of the sample but can indicate what it's made of and how
its atoms and molecules are organized. PEEMs can even
look beneath the surface to reveal magnetic structures.
Elements and their chemical environments are identified
and located by tuning the energy of the incident beam:
different energies (frequencies) stimulate electron emission
from different elements. If the x rays from the synchrotron
source are polarized, PEEM images can reveal the length
and orientation of chemical bonds, and -- depending on
how the beam is polarized -- depict the orientation of
magnetization in magnetic materials.
Aberrant behavior
 |
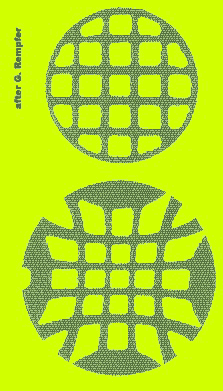
ELECTRONS BEND DIFFERENTLY NEAR THE EDGE OF A
LENS THAN NEAR ITS CENTER, RESULTING IN SPHERICAL
ABERRATION (BOTTOM). PHYSICIST GERTRUDE REMPFER
SHOWED THAT SUCH ABERRATIONS CAN BE CORRECTED (TOP)
WITH AN ELECTRON MIRROR.
|
Like an optical microscope, the spatial resolution of
a PEEM can be limited by aberration, so-called "image
error." Aberration also wastes electrons. Because
electrons ejected at the wrong angle or energy can't pass
through its focusing system, PEEM2 uses only about five
percent of the electrons emitted from a sample.
In an optical system like a camera, chromatic aberration
produces a rainbow effect when lenses bend light of different
colors (wavelengths) at different angles. Spherical aberration
produces fuzziness and distortion because a spherical
lens or mirror bends light rays at the edges more than
at the center.
Electrons behave in a similar way: different energies
focus at different distances from an electrostatic lens,
and electrons focus differently depending on their distance
from its center.
One way an optical camera can overcome aberration is
by correcting the convex lens (which focuses light rays
inward) with a concave lens (which spreads light rays
apart). But electrostatic lenses only focus inward. To
correct electrons, what's needed is a electron mirror.
The idea is to send electrons from the sample to a mirror
that can exactly compensate for aberrations and then send
the electrons back into the optical system. "Essentially
we want to reverse the sign of the defect and cancel it,"
says Feng.
Charged reflections
 |
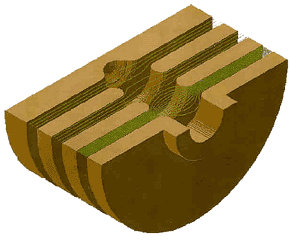
ELECTRIC FIELDS BETWEEN THE FOUR ELECTRODES OF
PEEM3'S ELECTRON MIRROR WILL CANCEL ABERRATIONS
IN THE BEAM AND REFLECT ELECTRONS BACK ALONG THE
PATH.
|
"The most advanced electron mirror so far was demonstrated
in 1997 by physicist Gertrude Rempfer at Portland State
University," Feng explains. "She proved that
a mirror can be used as an aberration corrector, but with
her two-electrode mirror, it's very difficult to cover
the whole range of aberrations."
The PEEM3 team is developing a four-element mirror mounted
at right angles to the main column of the microscope;
electric fields between the electrodes will cancel aberrations
in the beam and reflect electrons back along the path.
Design of the mirror and control of the voltages on the
electrodes depend on first accurately calculating all
aberrations in the electron path.
Scholl says, "The voltages can be adjusted within
a certain range, but from the viewpoint of the experimenter
it's really a tricky proposition, because you have little
more than your image to judge from." As a result,
the PEEM3 designers are developing computerized schemes
to optimize electrode voltages.
Among the trickier steps are extracting the aberrant
beam and returning the corrected one. An optical system
would use a beam-splitter like a prism, but to separate
and recombine its electron beam the PEEM3 will use a separator
magnet, inside of which the electrons loop around a complicated
path and are finally deflected by 90 degrees.
"We have several accelerator experts on our design
team, including David Robin and Ying Wu, and their expertise
in steering and conditioning charged particle beams is
invaluable," says Feng.
Thanks for your support
Crucial to the PEEM3 effort is a synchrotron beam with
an extremely high flux, whose energy can be concentrated
within the microscope's field of view and whose polarization
can be controlled. Beginning this fiscal year, the Department
of Energy's Office of Science is funding construction
at the ALS of a $3.8 million, elliptically polarized undulator
beamline. Critical supporting evidence for the beamline
proposal came from the initial design of PEEM3, funded
by a Berkeley Lab Laboratory Directed Research and Development
Program. Collaborators from many research institutions
and industries are participating in the development of
the microscope itself. Taiwan's Synchrotron Radiation
Research Center (SRRC) -- the first third-generation light
source in Asia -- IBM's Almaden Research Center, and the
ALS program provide personnel, fabrication facilities,
and other contributions in kind; other agencies are expected
to fund further development.
In addition to Jun Feng, PEEM3 researchers include Simone
Anders of IBM; Etienne Forest of Japan's High Energy Accelerator
Research Organization, KEK; Howard Padmore, leader of
the ALS's Experimental Systems Group; David Robin of Berkeley
Lab's Accelerator and Fusion Research Division (AFRD);
Michael Scheinfein of Arizona State University; Der-Hsin
Wei of SRRC; and Ying Wu of AFRD. Members of Berkeley
Lab's Engineering Division include Robert Duarte, Nicholas
Kelez, and Ross Schlueter. Andreas Scholl of ALS and Hendrik
Ohldag of Stanford work with the team in a consulting
capacity.
Additional information:
|