Early next year the National Institute of Standards and Technology (NIST)
will begin funneling almost $2 million into an effort originating at
Berkeley Lab to find a way of separating oxygen from air and pressurizing
it in a single step. The new, low-power electrolytic system will be
designed to produce oxygen right where it is needed -- in welding shops
and other light industries, for example, and in hospitals.
 |
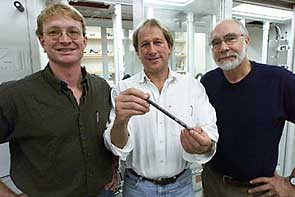
LEFT TO RIGHT: CRAIG JACOBSON, STEVE VISCO, AND LUTGARD DE
JONGHE DEVELOPED A THIN-MEMBRANE SYSTEM TO SEPARATE OXYGEN FROM AIR
|
The grant was made directly to Praxair, Inc., one of the world's
largest industrial gases companies, under NIST's Advanced Technology
Program, which supports high-risk research that companies can't fund
entirely on their own. In turn, Praxair is directing $1.2 million to basic
research and development by the oxygen system's originators, Steve Visco,
Craig Jacobson, Lutgard de Jonghe, and their colleagues in the Lab's
Materials Sciences Division.
One reason Praxair is interested in new ways to produce oxygen onsite,
says Visco, is that "for every pound of oxygen they deliver, they're
also delivering five pounds of steel." Oxygen, worth many billions of
dollars a year to the U.S. economy, is typically delivered in pressurized
steel bottles or as a bulk liquid or gas in tube trailer trucks.
Oxygen is also produced at the point of use. On a large scale, as in
the steel and glass industries, huge refrigeration plants liquefy air and
separate the constituents. On a smaller scale, vacuum-pressure swing
adsorption (VPSA) plants can be used even for home care, but "the
large PSA units in hospitals are very noisy and vibrate a lot, and the
concentrated oxygen is not pure," Visco says.
In search of a quiet, self-contained unit that can cheaply produce pure
pressurized oxygen electrochemically instead of mechanically, Praxair and
Berkeley Lab entered into a three-year cooperative research and
development agreement (CRADA) to adapt the solid-oxide fuel-cell work of
Visco, Jacobson and De Jonghe.
"We met Praxair representatives at a fuel-cell conference a few
years ago," says Visco. "An important part of our work on
solid-oxide fuel cells involved research on oxygen separation membranes,
and we were getting excellent performance."
"Like a solid-oxide fuel cell, the oxygen separation system is a
kind of ceramic sandwich," says Visco. "A porous ceramic
electrode supports a thin electrolyte membrane and another ceramic
electrode layer. In this case, however, we aren't supplying air and fuel
and producing electricity; instead we're supplying air and electricity and
getting pure oxygen."
Air, a mixture of gases about one fifth oxygen, flows through a porous
cathode and interacts with the electrolyte membrane, where the oxygen
atoms are charged by the current. These ions are drawn through the anode,
and molecules (paired atoms) of oxygen are reformed on the other side.
Visco, De Jonghe and chief technician Craig Jacobson investigated
various materials under the CRADA. In a novel approach, Jacobson
fabricated cathodes from a nonreactive ceramic, lanthanum strontium
manganite (LSM). Thin membranes of ytria-stabilized zirconia (YSZ) formed
the electrolytes, and anodes were made of a 50-50 mix of both these
compounds. Jacobson also devised a way to infiltrate dopants into the
ceramics to improve their electrical performance.
The easiest structures to fabricate were planar cells, which
demonstrated stable and efficient oxygen separation for up to a thousand
hours. But to capture the oxygen and pressurize it in a single step, the
researchers molded tubular cells, with the anode -- the so-called
"air electrode" -- on the outside and the electrolyte and
cathode applied in layers inside.
The tubular cells were heated to 800 degrees Celsius for efficient
electrolysis. As oxygen collected inside the tube, pressure increased; in
the CRADA tests, pressures reached 90 pounds per square inch.
When the CRADA ended this year, all its research goals had been met.
However, "if we are going to demonstrate a commercially viable
technology, we need to improve cell performance by an order of
magnitude," Visco says. "We need to develop stronger cells, so
we can compress oxygen to higher pressures, and we need to get the
temperature down to 700 C or less -- about the temperature of the wires
inside a kitchen toaster."
Under the new grant, the tubular cells Visco and his team are
developing are intended to produce a hundred milliliters of oxygen a
minute. Each about the size of a long cigar, many of them will be stacked
together to build a unit capable of reaching 50 to 100 liters a minute.
Devising the gas manifold and seals will be formidable tasks, to be
tackled by other research partners of Praxair.
One of the most interesting challenges facing Visco and his colleagues
is cell strength; one approach is to build the cell with a support
electrode of metal instead of LSM ceramic. "We're hopeful, but we
haven't overcome the challenges yet," Visco says. "That's why
NIST funds this kind of research."
To build a competitive oxygen separator, it won't be enough to
eliminate the need for steel containers and noisy or dangerous onsite
machinery. The bottom line is cost. For example, a competing separation
system based on ceramic membranes requires pressurizing the air to drive
it through the ceramic, as well as pressurizing the resulting oxygen, and
"it's expensive to compress high-purity oxygen," Visco remarks.
Nevertheless, Visco says, "the area of thin membrane separation is
really taking off. For years we were getting great results in the lab, but
finding money to support the research was both frustrating and
difficult." With NIST's Advanced Technology Program grant to Praxair,
he says, "support is finally coming in." |