BERKELEY, CA — Researchers in
the laboratory of Alexander Pines, a member of the Materials
Sciences Division of Lawrence Berkeley National Laboratory
and a professor of chemistry at the University of California
at Berkeley, have recovered high-resolution nuclear magnetic
resonance (NMR) spectroscopy data from experimental samples
in a grossly nonuniform field.
NMR pioneer Pines worked with postdoctoral fellow Carlos
Meriles and their colleagues Dimitris Sakellariou, Henrike
Heise, and Adam Moulé to develop the technique, which
could significantly extend the use of NMR spectroscopy
as an analytical tool. Their new "ex situ" method
is described in the 6 July issue of the journal Science.
Until now, high-resolution spectroscopy could only be
done "in situ," by placing the sample inside
the bore of a very large stationary magnet that produces
a strong, uniform magnetic field. With the new technique
it may soon be possible to take an NMR probe to otherwise
inaccessible samples in the field and obtain high-resolution
information.
"What makes NMR useful is that can provide a profile
of a sample, a kind of fingerprint," says Carlos
Meriles, "in which each component has recognizable
features." NMR spectroscopy has been used to study
the molecular structure and chemical dynamics of a vast
range of compounds, materials, and processes, everything
from an organism's metabolic state to the composition
of promising new materials.
The recognizable features in an NMR spectrum show up
as distinct peaks of varying height. Until now, in order
to clearly resolve two or more separate peaks, the sample
had to be placed in a static, uniform magnetic field.
Those nuclei in the sample with magnetic moments align
their spins, up or down, with the static field. When the
sample is irradiated with a radio-frequency (rf) pulse
matching the slight energy difference between the up and
down spins, it's as if the nuclei are knocked off balance,
precessing (wobbling) on a tilted axis around the field
lines.
Each species of nucleus has a characteristic wobble rate,
information that is reemitted as the nuclei relax and
realign with the static field. Because the same kinds
of atoms may experience different magnetic environments
in the presence of other nearby atoms, their signals can
differ and point to different chemical arrangements.
 |
The NMR spectrum of trans-2-pentenal shows five
chemical-shift peaks of hydrogen after a single
rf pulse in a uniform static field (A), but these
blend together in a nonuniform field (B). When static
and rf nonuniform fields are matched, the peaks
can be recovered by a train of specially tailored
pulses (C).
|
For example, signals from hydrogen nuclei in organic
compounds show up as different peaks in an NMR spectrum
depending on how the hydrogens are bonded to carbons or
other atoms. Displacements from a reference peak are called
"chemical shifts" and reflect different concentrations
of arrangements of hydrogen in the compound -- producing
a spectrum that positively identifies the specific compound.
"This way you can tell the difference between, say,
oil and water," says Meriles -- just the sort of
distinct signatures an oil-well logging instrument looks
for in a borehole. In fact, NMR probes have been devised
that can be lowered into boreholes, but they necessarily
produce uneven magnetic fields, which limits the information
they can gather.
In a nonuniform magnetic field, slight differences in
the magnetic environment of nuclei in one part of a sample
are overwhelmed by the much larger difference in static
field strength between different locations in the sample.
The peaks of different chemical shifts become so broad
they blend together, and the spectrum becomes featureless.
Once decayed, an NMR signal can be "refocused"
by zapping the sample with a second radio-frequency pulse.
This produces a so-called Hahn echo, a signal strong enough
to detect above the background. But methods based on the
Hahn echo erase any spectroscopic information.
"The signal still contains information -- it isn't
lost, but it's jumbled together," Meriles says. "All
you can say is, 'I've got a signal,' not whether it's
oil or water, for example." Attempts to get around
this roadblock have included analyzing various aspects
of the single smeared peak, although "interpretation
is extremely qualitative," says Meriles.
When Meriles and his colleagues in the Pines lab group
set out to solve the problem, they knew one key would
be to arrange matters so that the nonuniform static field
and the much weaker rf field both fell off smoothly in
a correlated way. They did this in the laboratory by imposing
a strong spatial variation on the static field, with the
rf coil placed at one end of the sample -- thus simulating
the conditions of a mobile, ex-situ experiment.
To visualize how lost spectroscopic information is recovered,
it helps to plot the chemical shift of each spinning nucleus
on a three-dimensional graph in which the static field
is oriented on the z (up and down) axis, and the rf field,
which varies regularly, is thought of as rotating in the
x-y plane. Viewed in a frame that rotates at the same
frequency, each distinctive chemical shift can be represented
by a vector in the x-y plane, rotating around the z axis
with that frequency. But in a nonuniform magnetic field,
spins get faster or slower, spreading the signal until
it overlaps other chemical shifts.
The researchers realized that the sharpness of the chemical-shift
vectors could be restored if the slow and fast offsets
could be exchanged, so that as the signal evolves these
components would converge, not spread. To do this they
designed a special sequence of pulses of precise energy,
duration, and timing.
The decaying NMR signal is zapped in such a way that
the first rf pulse lifts the vector out of the x-y plane,
where it is vulnerable to a second pulse timed to reverse
its previous dephasing; a third pulse equal to the first
lays the vector back down in the x-y plane but with fast
and slow spin segments now reversed.
Thus from a decaying signal that might otherwise smear
to featurelessness as it evolves in a nonuniform field,
the researchers are able to recover and intensify individual
chemical shifts to yield a high-resolution NMR spectrum.
The Pines group tested their arrangement on a series
of compounds, concluding with a liquid known as trans-2-pentenal,
whose characteristic spectrum, obtained with a single
rf pulse in a uniform static magnetic field, shows the
chemical shifts of hydrogen nuclei as five sharp spikes.
The same sample, if subjected to a single rf pulse in
a nonuniform field (outside the magnet bore), resembles
a featureless mound.
But if the sample is then subjected to the specially
tailored string of pulses in the same nonuniform field,
the five peaks are restored to their characteristic positions
and amplitudes on the spectrum, with virtually the same
sharp resolution.
"We have demonstrated that high-resolution NMR spectra
can be recovered even with a strongly inhomogeneous magnetic
field," says Meriles, "which means it may be
possible to develop a mobile magnet that can be scanned
over otherwise inaccessible objects to get magnetic resonance
information."
There is much to be done, he stresses. "You need
a really strong field to get a decent decay rate. The
stronger the gradient, the worse the problem. It's a challenge
to develop a strong magnet that can be taken into the
field, or to develop ways to recover a signal from a weak
field." But the principle of ex situ NMR spectroscopy
has been demonstrated.
"Approach to high-resolution ex situ NMR spectroscopy,"
by Carlos Meriles, Dimitris Sakellariou, Henrike Heise,
Adam Moulé, and Alexander Pines, appears in Science,
6 July 2001.
The Berkeley Lab is a U.S. Department of Energy national
laboratory located in Berkeley, California. It conducts
unclassified scientific research and is managed by the
University of California.
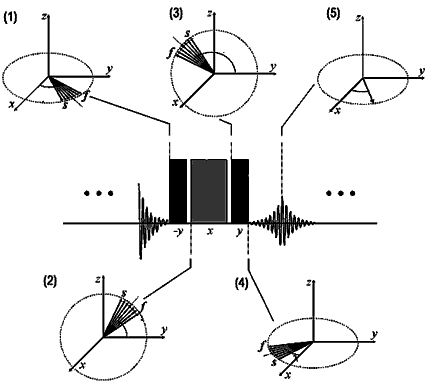
In a nonuniform magnetic
field, the chemical shift of hydrogen, plotted as a vector
in the x-y plane (1) has slow and fast spin components
(marked s and f) which spread the signal. A sequence of
pulses lifts the vector into the y-z plane (2), reverses
its previous dephasing (3), and lays it down again in
the x-y plane (4), with fast and slow components now reversed.
The chemical shift is subsequently recovered and intensified
(5).
Additional information:
|