BERKELEY, CA --
A clever modification of atomic-force microscopes, which are standard equipment in many laboratories, allows high-resolution images to be
made of a world never before seen in intimate detail: the surfaces of liquids.
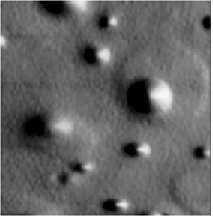
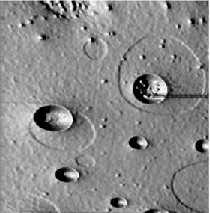
Salmeron's team has used the new microscope to make spectacular images of sulfuric acid corroding an aluminum surface. At top, the microscope is used in scanning polarization force mode. It shows liquid domes over flat "pancakes" of liquid. In the bottom image, the microscope's probe tip was allowed to descend, to touch the surface in atomic-force mode, revealing the shallow craters under the liquid pancakes. Beneath the standing drops, deep pits penetrated the salt to the uncorroded aluminum below. Such unsuspected details of corrosive reactions are pertinent to the acid-rain corrosion of airplane parts and other aluminum structures.
|
Ernest Orlando Lawrence Berkeley National Laboratory's Miquel Salmeron, who devised the technique, has recently used it to produce direct visual proof that crystals of ice are coated with a layer of liquid water, even at temperatures below freezing.
Salmeron, a member of the Lab's Materials Sciences Division, developed what he calls the "scanning-polarization-force microscope," or SPFM, to investigate liquid films and droplets with dimensions measured in a few tens of nanometers (billionths of a meter). Before the advent of Salmeron's SPFM, images of liquid surfaces were limited to what one could see with an optical microscope; the SPFM allows image resolution hundreds of times better.
"For a long time we've been able to see the surfaces of solids at very,
very high resolution," Salmeron says. "In fact we can make images of individual
atoms. But most atomic-level microscopy techniques require a probe to be in
contact -- or near contact -- with the surface. If you try that with liquids,
the tip sinks in, or the liquid wets it and climbs up, distorting the surface
you're trying to study."
In conventional scanning-tunneling and atomic-force microscopes, the tip
of a sharp probe scans across the surface of a solid substrate in near-contact.
What's in "contact" here are the electric fields of the atoms on the probe's
tip and those of the atoms on the surface. In scanning-tunneling microscopy, a
small voltage difference is established between the probe and the substrate,
creating an electric current; as the probe moves over the surface, the current
varies in strength according to the "shape" of the surface's electron clouds.
In atomic-force microscopy, the interaction force moves the tip, which is
mounted on a lever, up and down.
"Our trick was to lift up the probe to prevent distortion of the liquid
surface," says Salmeron. "There is a penalty for this, however -- the lateral
resolution is not better than the distance between the probe and the surface.
Still, that's only a few hundred angstroms, far better resolution than an
optical microscope, which is typically a few micrometers. And the height
resolution, which is under an angstrom, remains as good as in any other
scanning-probe microscope."
In another departure from usual practice, Salmeron chose not to use
electrically conductive samples, but to use insulating samples instead. Because
the electric field near the tip of the probe is determined mostly by its radius
and by the dielectric properties of the sample (a dielectric substance is one
that, compared to a metal, has low electrical conductivity), the other
electrode can be located far away, for example under a glass slide. "This
well-known effect was not fully appreciated previously," he remarks. "It opens
many possibilities."
The probe of the SPFM is a fiber of silicon nitride whose tip is thinly
coated with platinum, to which a few volts is applied. A laser beam, focused on
the back of the flexible fiber, is reflected to a detector diode, which records
the cantilever's dips and bends as the tip flies over the surface of the
liquid.
Two factors influence the resulting image. One is the distance of the tip
from the surface of the liquid. The other is the polarizability of the liquid
itself. In this way the SPFM not only produces a topographical map of the
liquid surface but can reveal changes in its dielectric constant. These may be
due to chemical properties or to ions moving in the liquid, or to trapped,
electrically polar molecules, as in water ice -- or to other factors yet to be
explored.
Salmeron and his coworkers have used the SPFM to make spectacular images
of sulfuric acid corroding an aluminum surface. The reaction of acid and metal
creates a salt, which in dry air precipitates on the uncorroded aluminum,
resembling a basaltic flow on the moon; within this moonlike "mare,"
liquid sulfuric acid fills deep craters. Both the liquid drops and the
underlying craters are visible in successive, registered images.
To make these images the microscope was first used in scanning-polarization-force mode, showing liquid domes over flat "pancakes" of liquid -- like egg yolks standing in whites, sunny-side-up. Then the probe tip was allowed to descend, to touch the surface in atomic-force mode, revealing the shallow craters under the liquid pancakes. Under the standing drops, deep pits penetrated the salt to the uncorroded aluminum below. Such unsuspected details of corrosive reactions are pertinent to the acid-rain corrosion of airplane parts and other aluminum structures.
"With the SPFM we can reexamine, at unprecedented resolution, many
macroscopic concepts about the interaction of liquids and solids," says
Salmeron. "A few that come to mind: atmospheric chemistry, involving not only
pollution but natural phenomena such as volcanic eruptions -- ozone depletion
-- the nucleation of clouds on carbon soot, on blowing dust, on ice crystals --
the wetting of cellular membranes . . ." and many others.
Salmeron's group has both confirmed theoretical predictions and found some
surprises. They have made images of water adsorption on ionic crystals such as
table salt (sodium chloride) and followed the microscopic process of crystal
dissolution. One study shows that fresh-cleaved sheets of mica exposed to air
over time exhibit increasingly branching patterns of wetting. In another study,
strings of nanometer-scale potassium hydroxide droplets persist along graphite
ledges only a few atoms high, even after the graphite has apparently been blown
"dry."
At the October, 1997 national meeting of the American Vacuum Society in
San Jose, California, Salmeron's group presented images of liquid water films
on ice crystals at temperatures well below freezing, one of many new phenomena
the invention of the scanning-polarization-force microscope makes accessible.
With a few deft modifications, any lab with an atomic-force microscope can
enter a new world of high-resolution images of liquids.
Salmeron obtained a patent on the SPFM earlier this year. Studies of water
adsorption on ionic crystals were reported in the Journal of Physical
Chemistry, vol 101, issue 11, March 1997. The SPFM's principles and the
results of several other studies are reported in MRS Bulletin, vol XXII,
no 8, August 1997.
The Berkeley Lab is a U.S. Department of Energy national laboratory
located in Berkeley, California. It conducts unclassified scientific research
and is managed by the University of California.