Researchers with Berkeley Lab’s Physical Biosciences
Division (PBD) have for the first time successfully unfolded
and refolded single molecules of RNA. By applying stretching
forces to molecules featuring one of three representative
RNA substructures, the researchers were able to observe
the molecules unfold as they would in a living cell and
measure the energy required to drive the folding reaction.
These experiments and the results hold importance for,
among other applications, the future design of antiviral
and other therapeutic drugs.
 |
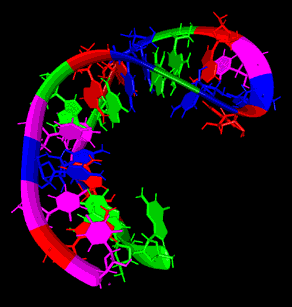
DEPICTED HERE IS A FOLDED "HAIRPIN," ONE OF THE
THREE TYPES OF RNA SUBSTRUCTURE EXAMINED BY RESEARCHERS
|
RNA -- ribonucleic acid -- is the workhorse of the genetic
world, transcribing the coded instructions of DNA and
assembling amino acids into proteins. What enables RNA
molecules to carry out their many biological tasks is
the ability of their nucleotide strands or helices to
fold themselves into complex three-dimensional structures.
Learning about the forces that drive and shape this folding
-- by unfolding RNA molecules -- is a key to designing
drugs that can enhance or inhibit the performance of a
specific task. For example, retroviruses such as HIV are
nothing more than protein-coated packets of RNA molecules.
"Traditionally, scientists have tried to unfold
RNA by temperature melting or by denaturing the molecules
with chemicals," says Carlos Bustamante, a member
of the experimental team who holds a joint appointment
with PBD and UC Berkeley (UCB) and is a Howard Hughes
Medical Institute (HHMI) investigator.
"The problem with those approaches is that they
were measuring massive numbers of molecules at a time
and averaging over this vast population. Add to that the
problem that every molecule might take a different pathway
to unfolding."
Says team member Ignacio Tinoco, "By pulling on
the ends of an RNA molecule, we are unfolding it more
like it may happen in the cell. As we learn about the
different paths for unfolding and refolding RNA molecules
we will also learn about transient RNA species that may
be good drug targets themselves."
 |
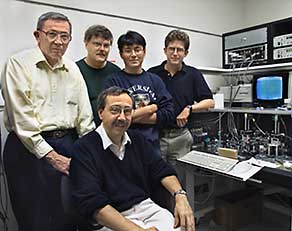
RESEARCH TEAM MEMBERS INCLUDE CARLOS BUSTAMANTE
(SEATED) AND (FROM LEFT) IGNACIO TINOCO, STEPHEN
SMITH, BIBIANA ONOA, AND JAN LIPHARDT
|
Adds Jan Liphardt, another team member, "This is
the first study in which the energetics of a three-dimensional
RNA structure were investigated under the physiological
conditions of temperature and ionic strength."
Like Bustamante, Tinoco and Liphardt also hold joint
appointments with PBD and UCB. These three were joined
by UCB researchers Bibiana Onoa and Steven Smith as co-authors
on a paper published in the April 27, 2001 issue of the
journal Science entitled "Reversible Unfolding
of Single RNA Molecules by Mechanical Force." This
work was done in part through UCB’s Health Sciences Initiative
which sponsors collaborative research to address major
health issues.
In their Science paper, the research team describes
how they were able to unfold and refold single molecules
of select RNA structure using a unique force-measuring
"optical tweezers" set-up that was designed
and built in Bustamante's laboratory. In this set-up,
an RNA molecule is tethered between two micron-sized polystyrene
beads in the middle of a chamber, one bead attached to
the tip of a piezoelectric actuator and the other anchored
by a laser beam. While the laser beam trapped and held
one end of the molecule, the piezoelectric actuator pulled
on the other end, causing the molecule to be stretched
out to the point where it unfolded. The research team
measured both the force required to unfold the molecule
and the changing length of the molecule as it was stretched.
Says Bustamante, "This system eliminates both the
problems of averaging large numbers of molecules and the
multiple reaction pathways because when we are pulling,
we are following a single molecule unfolding along a particular
pathway."
Because the major structural units or domains that make
up RNA molecules are relatively independent, it’s possible
to synthesize different types of domains and pull on each
to understand its distinctive characteristics. The three
types of RNA domains examined in this study were a folded
"hairpin," one of the most simple and common
secondary RNA domains; a "helix junction," another
common but more complicated secondary domain; and a molecule
that forms a tertiary RNA domain, a compact "bulge"
in which several secondary structures interact.
Both the hairpin and helix junction domains exhibited
a phenomenon called "hopping." This occurs when
molecules, held at a constant force sufficient enough
to allow transitions between the folded and unfolded states,
begin to hop back and forth between these two states.
From that hopping behavior, the Berkeley researchers were
able to measure the forces required to unfold the molecules,
plus their rates of unfolding and refolding and the energy
expended during the process. They found that the unfolding
forces coincided with the refolding forces.
"This means the process can be carried out at equilibrium,"
says Bustamante. "All the mechanical work we do to
pull the molecule is going to just break the bonds in
the molecule that maintain the folding."
The RNA domain with the bulge displayed an unfolding
phenomenon called "ripping." This occurs when
the molecule partially unfolds and then pauses. Only upon
a slight increase in the pulling force will the molecule
abruptly unfold the rest of the way.
"When you start looking at even more complex domains,
you start seeing different pathways, with similar parts
of the molecule unfolding at different forces," Bustamante
says.
The scientists also investigated the folding characteristics
of RNA domains in the presence of magnesium ions which
are known to be necessary for RNA to carry out its biological
functions. They found that when an RNA molecule achieved
its three-dimensional structure in the presence of magnesium
it became much more difficult to unfold.
Says Tinoco, "I was surprised to learn just how
important magnesium ions are to the unfolding of RNA molecules.
We found that the main effect is on the kinetics of unfolding
rather than on the energy needed to unfold the RNA."
Ultimately, the Berkeley researchers, through these single
molecule mechanical unfolding and refolding experiments,
would like to be able to provide biochemists with an energy
function curve that would identify the energy barriers
required to hold a given RNA molecule together and the
force needed to unfold it.
Explains Liphardt, "Once you know this energy function
curve, you will know (for a given RNA molecule) something
about how your molecule folds, its most stable conformation,
and the alternative conformations it can explore at a
given temperature. You’ll also know something about how
it might deform when it interacts with other molecles,
such as enzymes or drugs. If you’re a biochemist trying
to design an RNA molecule with a particular folding pathway
and final conformation this information would help."
Members of research team say their results so far are
merely a hint of the wealth of data on RNA folding that
their experimental technique can provide.
|