|
 |
BERKELEY, CA — In the race to stay one step ahead
of drug-resistant bacteria, scientists from Lawrence Berkeley National
Laboratory and the University of California at Berkeley obtained high-resolution
images of a protein complex found in bacteria that repels a wide range
of antibiotics.
The images, which appear in the May 9 issue of Science, offer new
insight into how bacteria survive attacks from different antibiotics,
a growing health problem called multidrug resistance. As the team learned,
these robust defenses are rooted in the protein complex’s remarkable
ability to capture and pump out a spectrum of structurally diverse compounds.
The research may inform the development of antibiotics that either evade
or inhibit these pumps, allowing drugs to slip inside bacteria cells and
kill them.
 |
|
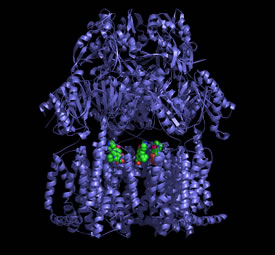 |
|
Even ciprofloxacin, an antibiotic used to treat
a variety of bacterial infections including inhaled anthrax, is no
match for AcrB. In this image, the green-colored drug is firmly ensnared
in the protein’s cavity. |
|
The team focused their inquiry on AcrB, a protein that resides in the
inner membrane of Escherichia coli cells. It works in unison with
two other proteins to rid the bacteria of toxins. Based on earlier research,
they knew AcrB boasts a large cavity capable of binding with a vast range
of antibiotics and other molecules. But precisely how this cavity accommodates
so many shapes and sizes remained unclear. To witness this trickery, the
team crystallized the protein in the presence of four molecules —
an antibiotic, a dye, a disinfectant, and a DNA binding molecule —
and then turned to Berkeley Lab’s Advanced Light Source (ALS). There,
they exposed the crystals to extremely bright x-rays that reveal the protein’s
molecular structure, including how the four molecules bind to the cavity.
The resulting images portray a hungry, indiscriminate binding site. Each
molecule bound to different locations in the cavity, and each bond utilizes
a different set of amino acid residues. And there’s probably room
for many more types of interactions.
“The protein has a very promiscuous binding mechanism,” says
Gerry McDermott, a staff scientist in Berkeley Lab’s Physical Biosciences
Division.
The 3.5 to 3.8-angstrom-resolution images (one angstrom equals one ten-billionth
of a meter) provide the closest look yet of a phenomenon common to all
living cells: the ability to expel a diverse flotilla of toxins using
one pump. In healthy people, these so-called multidrug efflux pumps play
important roles. A complex called P-glycoprotein, for example, prevents
the entry of toxic molecules across the mucosal surface of the intestinal
tract, as well as the blood-brain barrier. But P-glycoprotein has a dark
side. When overexpressed, it makes cancer cells resistant to a wide range
of chemotherapy drugs.
A similar split personality is seen in E. coli and its all-purpose
pump. On the good side, harmless colonies of the bacteria inhabit animal
intestines. In this environment, scientists theorize AcrB’s chief
function is to trap and pump out toxic bile salt. Unfortunately, a mutated
form of E. coli causes food poisoning. And, making matters worse,
AcrB shields the bacteria from many more compounds in addition to bile
salt, which explains why a broad regimen of antibiotics is needed to fight
the infection.
“The protein is a bit too efficient for our own good,” McDermott
says. “And our work demonstrates why. We suspect the protein’s
cavity possesses areas where many types of antibiotics could be captured.”
Their research also underscores the need to pursue alternative ways of
fighting drug resistance. Currently, pharmaceutical researchers combat
resistance by tweaking an antibiotic’s molecular structure so it
isn’t compatible with a pump’s binding site. This is difficult
work to begin with, largely because antibiotics must adhere to strict
potency and safety regulations that limit the extent to which they can
be modified. Add to these restrictions a better understanding of AcrB’s
readiness to bind with an array of compounds, including the most carefully
engineered antibiotics, and the job appears even more difficult.
“Our work demonstrates that changing antibiotics to beat resistance
is not a trivial task,” McDermott says.
Instead, McDermott believes their research supports another strategy
in which the multidrug efflux pump is disabled. In E. coli, such
monkey wrenching is possible because AcrB connects to a funnel-shaped
protein embedded in the bacteria cell’s outer membrane. This protein
ejects the antibiotics trapped in the AcrB cavity. If it’s blocked,
the pump can’t work. As McDermott explains, perhaps a specially
designed molecule could lodge inside the funnel, rendering the pump useless
and allowing antibiotics to slip inside the cell unhindered.
To support these advancements in drug design, the team will next use
the ALS to more fully explore how AcrB binds with a structurally diverse
range of antibiotics. They believe a fuller understanding of E. coli’s
multidrug efflux pump will shed light on similar pumps found in other
harmful bacteria, which could lead to better treatments for a variety
of infections.
“Drug resistance has become a global problem,” McDermott
says. “Our research will help design drugs that reduce resistance
to antibiotics.”
In addition to McDermott, Helen Zgurskaya of the University of Oklahoma,
and Hiroshi Nikaido, Daniel Koshland, Jr., and Edward Yu of the University
of California at Berkeley contributed to the research. The x-ray crystallography
work was conducted at ALS beamline 8.2.2, which is funded by the Howard
Hughes Medical Institute.
Berkeley Lab is a U.S. Department of Energy national laboratory located
in Berkeley, California. It conducts unclassified scientific research
and is managed by the University of California.
|