The startling announcement in 1998 that the universe is expanding at
an accelerating rate followed not long after by evidence that a
mysterious "dark energy" filling the universe is responsible
for this acceleration was based on comparing the redshifts and
magnitudes of numerous Type Ia supernovae, exploding stars of extreme
and remarkably uniform brightness.
 |
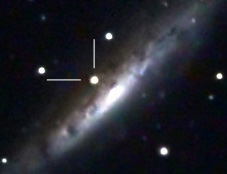 |
|
|
|
A Type Ia supernova discovered
in 2001 (Photo: Ted Dobosz). |
 |
|
The quality and quantity of data on these and other types of supernovae,
both at high and low redshifts, will increase exponentially in the next
few years because of two experiments based at Berkeley Lab: the well known
Supernova Cosmology Project and a newer program, the Nearby Supernova
Factory. Their goal is to determine the underlying physics behind these
catastrophic events, so that they can be used to understand dark energy
and other cosmological questions.
"The only way to fully exploit the power of the amazing data now
being collected will be to make an equally dramatic improvement in computational
studies of supernovae," says Peter Nugent, an astrophysicist in the
Scientific Computing Group at the National Energy Research Scientific
Computing Center (NERSC). "Unfortunately, current computational resources
only allow us to fully model supernova atmospheres in the spherically
symmetric regime."
Researchers have done their calculations as if supernovae explode spherically,
that is, the same way in every direction, in order to simplify computer
modeling. Recently, however, Nugent was awarded a three-year, $500,000
grant by NASA's Astrophysics Theory Program to help expand supernova modeling
to the third dimension. "The more we look at supernovae," he
says, "the more we realize that assuming they are 1D explosion events
is a horrible oversimplification, which might lead us down some very wrong
paths concerning the nature of their progenitors and their explosion mechanisms."
Nugent will take advantage of coming increases in the computational power
of NERSC's massively parallel supercomputers, as well as improvements
in the algorithms used to calculate supernova hydrodynamics and processes
like radiative transport.
"We'll be able to put tight constraints on the characteristics of
supernova progenitor stars, including their luminosities, explosion mechanisms,
and nucleosynthesis products, once we can compare computed spectra based
on different progenitor models with high-quality observations from the
Supernova Cosmology Project and Nearby Supernova Factory," Nugent
says.
Modeling will also address other effects, such as the possibility that
different compositions of the progenitor star, owing to the evolutionary
stage of the universe when the star formed, might somehow bias the determination
of cosmological parameters.
Type Ia supernovae in particular are complex events. They begin as white
dwarf stars made of carbon and oxygen, accreting a companion star's material.
Eventually they accumulate a critical mass, 1.4 times the mass of the
sun: deep within the white dwarf, carbon fuses, setting off a runaway
thermonuclear explosion. Despite whatever differences there may be among
progenitor systems, the critical mass is the same in all cases, so Type
Ia supernovae have very similar spectra and rising and falling light curves.
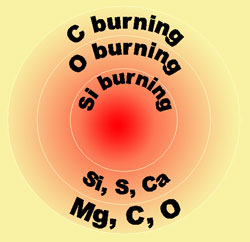 |
|
|
|
Type Ia supernova explosion creates an abundance
of intermediate-mass elements, requiring a transition from subsonic
deflagration to supersonic detonation.
|
|
|
|
A Type Ia ejects material at velocities approaching 20 percent of the
speed of light and, unlike other types of supernova, produces a great
many elements of intermediate mass between carbon and nickel, which are
created at different densities. To account for this, different models
invoke scenarios of explosions involving different rates at which a relatively
slow-burning deflagration front makes the transition to a supersonic detonation
wave.
An important new source of information that could provide insight into
these processes is the supernova polarization data taken by Lifan Wang
and Andy Howell of Berkeley Lab's Physics Division. Different regions
of an exploding star's atmosphere may be moving at different rates or
in different directions; some regions may be richer or poorer in various
elements; viewed from a particular angle, the remnants of a companion
star or an accretion disk surrounding the progenitor may block or filter
some of the supernova's light. These details leave their mark as different
orientations of the polarized light in different spectral lines of the
exploding star.
"One of our basic goals is to start making models in 3D that exploit
the new polarization data," Nugent says. "Polarization allows
you to probe the geometry of the event."
While polarization data show that the geometries of other kinds of supernovae
vary more than Type Ia supernovae and that their differences persist longer,
a Type Ia can still reveal much about itself through polarization if it
is caught early. Despite their apparent similarity, Type Ia's are not
identical.
Already Nugent and graduate student Daniel Kasen have begun constructing
models that use polarization to explore supernova geometries, including
determining the angle at which these ancient cataclysms have been observed.
"For the first time we are learning details about supernova progenitors
from the data," Nugent says. "If what we're seeing proves out,
it may even create shockwaves right here in the supernova community."
|