|
 |
An untuned SQUID is not the whole answer to measuring NMR with extremely
weak fields. To detect NMR signals at all there must be net polarization,
an overall magnetization resulting from the existence of a few more "spin-up"
nuclei than "spin-down" nuclei. Even in high fields, spin-up
nuclei hold the majority by only about 1 in 100,000; the weaker the magnetic
field, the weaker the polarization.
 |
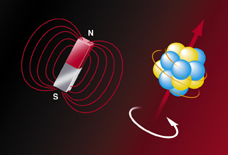 |
|
|
|
Some spinning nuclei have
magnetic moments not unlike toy bar magnets and orient
themselves with the field lines of an external magnetic field. |
|
|
The experimenters met the challenge by using two magnetic fields. One,
of about two millitesla, first prepolarizes the sample then is quickly
shut off. The measurement field at a microtesla, a thousand times
weaker is applied at right angles.
"We get the best of both worlds," Trabesinger remarks, "high
polarization from the higher field, plus narrow lines from the low measurement
field."
Abruptly turning off the polarizing field creates its own problem, however:
the precessing nuclei are pushed out of phase. To correct this, the measurement
field is reversed. Wandering precessions instantly reverse direction and
"wobble backwards" into maximum polarization.
A final disadvantage of low-field NMR is that chemical shifts, caused
by the screening effects of nearby electrons, vanish in microtesla fields.
But a different kind of nuclear signature, imposed by the quantum states
of shared electrons in chemical bonds, persists. This scalar coupling
(or J coupling), says Trabesinger, "is intrinsic. It doesn't depend
on the external magnetic field, so it doesn't change in a low field."
"Information about chemical bonds can be resolved even in incredibly
inhomogeneous magnetic fields," adds McDermott. Scalar coupling opens
the door to chemical sampling even when magnetic signals vary, as in living
organisms or the fluid-filled porous rocks measured in oil-well logging.
Spying on bonds
Unlike a traditional tuned NMR system, an untuned SQUID is "broadband"
it can simultaneously detect different kinds of nuclei precessing
at different frequencies. The Clarke-Pines team detected the nuclei of
phosphorus and hydrogen (protons) in the same measurement of phosphoric
acid.
Moreover, "through detection of chemical bonds, we can monitor chemical
reactions as they happen," McDermott says. At low fields the NMR
spectrum of phosphoric acid and methanol mixed with water shows only a
single proton peak, while the spectrum of trimethyl phosphate the
reaction product of phosphoric acid and methanol shows the proton
peak split in two by the scalar coupling of the compound's phosphorus-hydrogen
bonds. With labeled "spy nuclei," a low-field "bond detector"
might track the formation of other bonds like these.
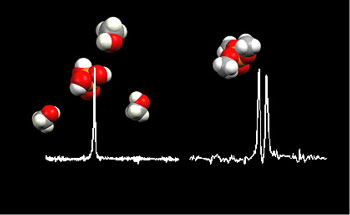 |
|
When mixed with water, phosphoric acid and methanol
show only a single proton peak in a low-field NMR spectrum, but
for trimethyl phosphate the reaction product of phosphoric
acid and methanol the proton peak is split in two by the
scalar coupling of bonds between phosphorus and hydrogen.
|
|
The low-field SQUID technique could also be used in MRI. Since the precession
rate of spinning nuclei depends on the strength of the external magnetic
field, their spatial distribution can be plotted to create an image. MRI
typically has depended on strong magnetic fields that fall off rapidly;
in medical diagnosis machinery, this has meant housing the magnet in a
large torus, into which the patient is slid on a table.
Not only are the big machines expensive and immobile, their high fields
are dangerous to patients with pacemakers or metal prosthetic inserts.
Moreover, Trabesinger remarks, "it's not easy to convince everybody
to get into these monsters. Some get a sudden attack of claustrophobia."
A low-field detector, on the other hand, could achieve high resolution
with a small field gradient, and the detector's portable small coil could
be moved over the patient. Among other advantages, this would allow patients
to assume a more natural posture. "There are lots of physiological
situations where lying down might not be the best position to measure,"
Trabesinger says, "for example, the stomach's position while swallowing."
Low-field NMR will never replace traditional high-field methods, because
only high fields make available the powerful diagnostic capabilities of
chemical shift. In the right circumstances, however, low-field NMR could
give limited but potentially very useful information about the scalar
coupling of many molecules.
One application that excites both McDermott and Trabesinger is the possibility
of studying the brain by combining MRI with direct detection of chemical
bonds, yielding a unique way to investigate the mysteries of brain chemistry.
"It would not be easy," says McDermott, "but the new low-field
SQUID detector may make this possible."
"Liquid-state NMR and scalar couplings in microtesla magnetic fields,"
by Robert McDermott, Andreas H. Trabesinger, Michael Mück, Erwin
L. Hahn, Alexander Pines, and John Clarke, appeared in the 22 March 2002
issue of Science.
Additional information:
|