To meet the demands of today's high-volume genome sequencing projects,
Berkeley Lab researchers have developed a powerful magnet that obtains
DNA samples much faster than commercially available magnetic plates.
The magnet, which borrows from more than a decade of research that informed
the development of particle accelerator magnets, can be applied to several
emerging biotechnology fields. In addition to gene sequencing, it can
be used in functional genomics, which is the study of what genes do. And
it can be used in proteomics, which is the study of how proteins both
activate and function in organisms.
It's based on a hybrid approach in which permanent magnets are coupled
to ferromagnetic materials. This combination produces magnetic fields
that are much stronger than those produced by magnet plates based solely
on permanent magnets, according to David Humphries of Berkeley Lab's Engineering
Division.
|
 |
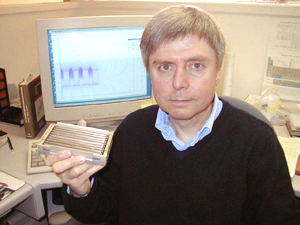 |
|
David Humphries with the hybrid magnet
he helped develop for biotechnology applications |
|
So far, Humphries and colleagues have used the magnet to more efficiently
conduct several sequencing initiatives at the Department of Energy's Joint
Genome Institute (JGI). In the lab, it's used in a common process that
draws genetic material out of solution. The technique uses hand-sized
plastic plates, called microtiter plates, that are pitted with 384 tiny
wells. Each well is filled with a solution that contains DNA. Next, tiny
magnetic particles measuring one micron (one millionth of a meter) in
diameter are added to the solution. These beads are coated with a carboxylate
layer that is specially chosen because it binds with DNA. In this manner,
the carboxylate-coated bead attaches to the DNA, and the DNA is magnetized.
Finally, the microtiter plate is placed on the magnet plate, and the DNA
is drawn to the bottom of each well and out of the solution.
Until recently, this process relied on relatively unsophisticated commercial
magnets that are adequate for small benchtop applications, but are hard-pressed
to accommodate high-volume processes in which millions of base pairs of
genes are sequenced. Such volumes are increasingly required in comparative
sequencing efforts in which the genomes of relatively simple organisms
are compared with the largely finished human genome. For example, the
JGI recently sequenced the sea squirt, which at one stage in its life
cycle possesses the most primitive notochord, or vertebral column, of
any animal. Understanding how this structure develops in sea squirts may
shed light on how it develops in humans.
To undertake these mind-bogglingly massive comparative genome studies,
engineers have developed sophisticated liquid handling robots that deposit
DNA-containing solutions into microtiter plates, and high-speed capillary
electrophoresis sequencing machines that read the DNA once its pulled
from the solution. However, the middle step -- drawing the DNA out of
solution -- often looms as the weak link because of slow, weak magnets.
That's where Berkeley lab's hybrid magnet comes in. It boasts a field
strength, or magnetic pull, that at its surface is 70 percent stronger
than the most powerful commercial magnet. And one centimeter above the
magnet, the magnet's pull is 400 percent stronger than commercial magnets
used with 384-well plates, and 1,000 percent stronger than commercial
magnets used with 96-well plates. This means the magnet quickly and thoroughly
grabs DNA floating near the top of even thick, viscous solutions. And
it securely holds DNA at the bottom of wells, especially important given
that liquid handling robots subject solutions to considerable turbulence.
|
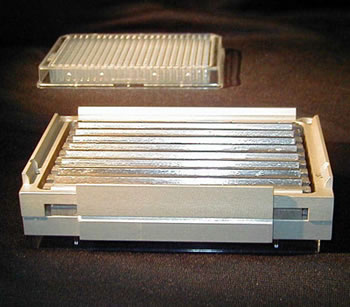 |
|
The hybrid magnet, foreground, attracts
strands of DNA attached to magnetic particles in a microtiter plate,
background. |
|
In addition, the magnet's gradient, or the change of its field strength
over distance, can be manipulated. This means magnetized particles can
be held at user-defined positions in the microtiter wells, enabling researchers
to selectively separate different particles from a solution.
Together, the magnet's improved field strength and gradient distribution
translates to unprecedented production rates. Industrial sequencing processes
are judged by a quantitative yardstick called read length, which is the
number of base pairs of genes that are sequenced in a single cycle. Of
equal importance is its pass rate, a qualitative assessment that measures
the amount of acceptable data obtained in the sequencing process. The
higher this percentage, the less data must be discarded and the more efficient
the technique. Today, reading 500 high-quality base pairs per cycle is
considered good. The JGI production sequencing process, using the hybrid
magnet, produces read lengths of 630 base pairs with pass rates above
90 percent.
"That's an industry milestone," Humphries says. "No facility
we know of comes close to these read lengths and this sample rate."
The revved-up magnets have helped double the JGI's sequencing capacity.
The lab's previous sample processing system employed 96-well microtiter
plates and pure permanent magnets. This highly automated system produced
roughly 20 million sequenced bases per day, a level that was one of the
highest of any sequencing laboratory.
The current sample processing system employs both 384-well microtiter
plates and the new hybrid magnet technology. Today, JGI researchers sequence
more than 40 million bases per day. In addition, the system's improved
efficiency has enabled the lab to reduce both the process's space and
personnel requirements.
Look for these sequencing rates to get even faster. The next step, according
to Humphries, is to tailor the hybrid magnets to fit next-generation microtiter
plates with 1,536 wells.
"The motivation behind these magnets is to sequence as fast as possible,"
Humphries says. "And although the initial goal of the human genome
project is largely accomplished, there is a tremendous amount of work
left to be done."
Berkeley Lab's Martin Pollard and Livermore Lab's Chris Elkin, along
with a number of JGI and Berkeley Lab employees, greatly contributed to
the technology's success.
|