Billions of barrels of oil lie in the Lost Hills and Belridge oilfields
in Kern County, right in the middle of California. The catch is, less
than ten percent of it can be extracted from the rock with current technology.
|
|
 |
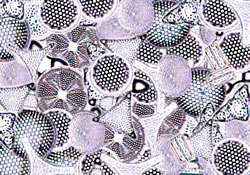 |
|
|
 |
Sinking to the bottom of shallow lakes
and seas, the glassy skeletons of unimaginable multitudes of microscopic
plants formed the remarkable rock known as diatomite. |
|
|
The rock in question is diatomite, made of countless silica skeletons
of microscopic aquatic plants. Diatomite's tiny pores range from a few
billionths to a few millionths of a meter wide, creating a soft and fragile
mineral that is up to 70 percent empty space -- light enough to float
on water but so nearly impermeable that no one is quite sure how the oil
got into the rock in the first place.
"The only way to get the oil out is to fracture the rock,"
says Tad Patzek of Berkeley Lab's Earth Sciences Division (ESD), who is
also a professor of civil and environmental engineering at UC Berkeley.
Patzek notes there was virtually no production from Lost Hills until 30
years ago, when the hydrofracturing technique -- breaking the rock with
pressurized water -- was first used. The ChevronTexaco Company currently
extracts some 10,000 barrels of oil a day in the Lost Hills Field alone.
One result of oil extraction was that parts of the oil field began to
collapse and subside. In 1992 ChevronTexaco introduced a program of balanced
waterflooding in the Lost Hills Field in hopes of reducing subsidence
and increasing oil recovery, but results have been mixed.
Patzek and his colleague Dmitry Silin of ESD are working with the ChevronTexaco
team, led by Jim Brink and Pat Perry, to find better ways to tap the rich
but miserly diatomite formation. They are pursuing a proposal by Grigory
I. Barenblatt of the Mathematics Department in Berkeley Lab's Computing
Sciences Directorate, a professor of mathematics at UC Berkeley and a
world-renowned expert in petroleum engineering and rock mechanics.
Barenblatt recently advanced a new concept of damage propagation in diatomite,
with consequent modification of rock properties; initial computer simulations
based on the model suggest new technologies for oil recovery. Recent work
has also benefited from startling new microscopic images of diatomite
made by Liviu Tomutsa of ESD and Velimir Radmilovic of the National Center
for Electron Microscopy (NCEM).
"To understand and predict what's happening in diatomite reservoirs,
the big picture is not enough," says Patzek. "We're tackling
the problem on the very small scale as well, trying to understand the
3-D structure of the rock itself."
Oil and water
When water is injected into an oil-bearing formation under pressure two
things happen -- at least in theory. The fractured rock is supposed to
release the oil, and the water is supposed to push it into flow-conducting
fractures, further toward the production wells. Meanwhile the water presumably
fills the voids left behind, maintaining the pressure and structural integrity
of the rock formation.
|
|
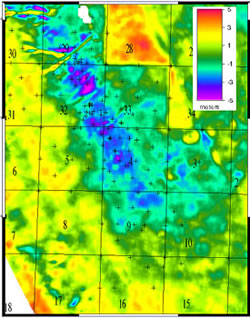 |
 |
|
|
Over a period of 17 years the surface
of the Lost Hills oilfield sank about three meters, as indicated in
blue and purple. Meanwhile fruit trees in a nearby orchard, sections
28 and 34, grew to heights of five meters, as indicated in red and
yellow. (Each section is about a mile square.) |
|
|
|
But even though waterflooding at Lost Hills has been carefully planned
and monitored, it has produced less than textbook results. Water injection
has damaged the rock in unexpected ways, causing the ground to subside
over wide areas -- in some regions, 10 feet or more -- and shearing off
well casings. Much of the water pumped down the injection wells comes
right back up the production wells without increasing the amount of recovered
oil.
"You can't produce from diatomite without injection, but we've got
to learn how to control the process," says Patzek. "On the large
scale we're looking at the overall structure of the formation."
One approach is to look at where and how the ground has moved and compare
that data with records of the water injected and the oil produced. InSAR
images, radar images from space satellites taken from different viewpoints
and combined interferometrically, graphically reveal ground subsidence
in the oil fields. Subsidence as oil is pumped out shows up immediately.
Waterflooding has done less than expected to stop subsidence, no matter
how much water is injected.
The reason lies in the geological complexity of the Lost Hills formation.
The diatomite beds are divided into discrete segments by vertical faults
and horizontal strata, with layers varying in thickness from less than
a millimeter to tens of meters. Not only can excessive water-pressure
crush diatomite, it can cause the weakly bound layers to break apart.
Channels open that carry water directly from injection well to production
well without moving any oil.
|
|
 |
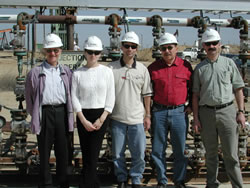 |
|
|
 |
A Berkeley Lab and ChevronTexaco team
designed and installed automatic injection equipment to maintain the
right water pressure in the Lost Hills diatomite. From left, Gennady
Goloshubin of the West Siberian Research Institute of Geology and
Geophysics, a guest of the Berkeley Lab researchers; Monika Valjak,
ChevronTexaco field engineer; Jim Brink, ChevronTexaco field advisor;
Tad Patzek and Dmitry Silin of ESD. |
|
|
To prevent the creation of channels and to keep soft diatomite from collapsing,
water injection pressures must be monitored and adjusted at frequent intervals.
Working with colleagues at ChevronTexaco, Patzek and Silin have developed
a software system that acquires pressure and flow-meter reading from Lost
Hills wells over a computer network. In automatic mode, the data are analyzed
and the results immediately sent back to the field.
Since February, 2002, the Supervisory Control and Data Acquisition (SCADA)
system at ChevronTexaco has controlled 12 automated water injectors in
real time. "The data is transmitted right here to my desktop PC,"
says Silin. Early results have been encouraging, and the company has budgeted
support for SCADA for coming years.
From macro to micro
Seeking a theoretical understanding of the structure and mechanical properties
of oil-bearing rocks, one based on fundamental physical principles, Silin
and Patzek, with their colleague Guodong Jin of the University of California
at Berkeley, have used computer modeling to study how sediments become
rocks in the first place.
|
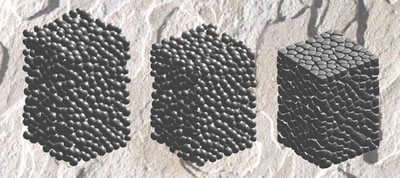 |
|
Model sandstone formation begins with
sedimentation (left), as grains of different sizes settle under the
action of gravity. Adding the pressure of overburden causes compaction
(center). Cementation (right) is one of the final processes that turns
sediment into rock. |
|
They begin with a collection of grains, idealized as spheres of different
sizes, randomly distributed as in natural sediments. These are then compacted,
as natural deposits are when deeply buried. They then model the process
of diagenesis by which the compacted grains become cemented together.
Finally they derive the mechanical properties of the resulting model rock.
Their model is unusual because it incorporates the dynamic processes
of sedimentation and compaction, including gravity, contact forces, friction
between grains and with the surrounding fluids -- all the ways that grains
of different size and composition may move and interact.
The program has produced astonishing model rocks. Its reconstruction
of sandstone is virtually indistinguishable from images of real sandstone
made by synchrotron X-ray tomography. Eventually computer reconstructions
may make it possible to generate realistic images and understand the mechanical
properties of any kind of sedimentary rock -- including diatomite -- at
will.
Picturing the real thing
While X-ray tomography can image relatively coarse sandstone at a resolution
of a few millionths of a meter (microns), the only way to characterize
the very fine pore structure of diatomite and chalk is at the nanometer
scale -- billionths of a meter.
ESD's Liviu Tomutsa has been working with NCEM's Velimir Radmilovic,
of Berkeley Lab's Materials Sciences Division, to produce remarkable pictures
of real reservoir diatomite. They have used focused ion beams to image
its invisibly tiny pores and connecting throats, the empty spaces that
constitute over half its volume.
|
|
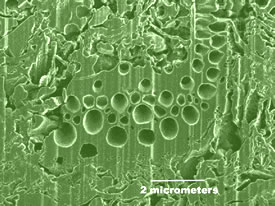 |
 |
|
|
A beam of gallium ions shaves off
a sample of diatomite layer by layer, imaging each layer. A computer
will combine the succeeding images to reconstruct the rock in three
dimensions. |
|
|
|
For some time NCEM has prepared samples for transmission electron microscopy
(TEM) with ion-beam milling. TEM images columns of atoms in a crystal
by sending a beam of electrons right through it, a kind of shadow picture.
For best results the sections must be extraordinarily thin, typically
a few microns or less. No mechanical slicing or polishing technique can
operate on so fine a scale without tearing the sample to shreds.
A sweeping beam of electrically charged gallium atoms, however, mills
a sample that is nearly perfectly flat. The ions do more: as they sweep
across the sample, sputtering off patches of atoms a few dozen at a time,
they free secondary ions that can be focused into a high-contrast image
of the surface at a resolution as fine as 10 nanometers.
"As the beam shaves off layers of material only a few nanometers
thick, it simultaneously makes a stack of 2-D images," says Tomutsa.
"These can be stored in a computer and combined into a 3-D picture
that reveals the constrictions and connectivity of the pores, the factors
that affect trapping of liquids."
Tomutsa and Radmilovic are now working to merge their individual 2-D
images into pictures with three-dimensional depth. Says Tomutsa, "These
will be the first-ever images of diatomaceous rock in 3-D." What's
more, he says, by using the ion beam to mill and image samples at very
low temperatures, "we'll actually be able to see the oil inside the
rock, undisturbed, frozen in the pores."
Computer modeling and real imaging may soon converge to understand the
structures of rocks on the finest scale, both theoretically and experimentally.
One result, says Tomutsa, could be a single number that expresses the
relative permeability of a particular kind of rock under many different
conditions.
It will be a dramatic step forward, giving energy companies a head start
on choosing the best techniques for extracting oil from tough formations
and relieving the expensive trial and error that characterizes oil recovery
today.
Additional information
|