|
 |
In a development that may lead to less expensive
drug production processes mediated by enzymes, Berkeley Lab chemists have
successfully replaced a natural compound used in biocatalytic reactions
with a simpler and more stable analog.
The team stripped a naturally occurring molecule used as a cofactor in
enzymatic reactions one with the jawbreaking name 1,4-dihydronicotinamide
adenine dinucleotide, more often called 1,4-NADH of its sugar,
phosphate, and adenosine groups. The simpler compound, called a biomimetic
because it mimics the more complex molecule, was then used instead of
its naturally occurring counterpart in a common catalytic cycle in which
an enzyme produces a pharmaceutically important molecule called a chiral
compound.
 |
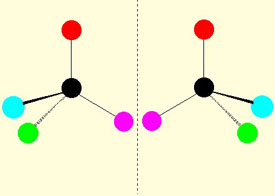 |
|
|
|
Enantiomers are mirror-image molecules
that are structurally equivalent but cannot be superimposed because
of their "handedness," or chirality. Also called optical
isomers, in pure solutions one of a pair rotates the plane of polarized
light to the right, the other to the left. |
 |
|
Chirality refers to the "handedness" (right or left handed)
of molecules which occur in two forms, mirror images which cannot be superimposed
upon each other. Such molecules are called enantiomers. Chiral compounds
used in the pharmaceutical industry are characterized by their optical
purity as single enantiomers overwhelmingly right handed or left
handed a critical requirement in large-scale drug production processes.
"We fooled the enzyme into thinking our stripped-down, reduced cofactor
mimic is the natural compound," says Richard H. Fish of Berkeley
Lab's Environmental Energy Technologies Division. "This marks the
first time, as far as we can tell, a biomimic of 1,4-NADH has been used
to produce a chiral compound."
The team's success, although still in the experimental stage, promises
to streamline a sometimes expensive drug production method using enzymes.
In this process, enzymatic reactions produce chiral molecules of one optical
form. Drug manufacturers favor this enzyme-based method over processes
that rely on synthetically formulated chemicals because enzymes require
less energy to perform reactions. Millions of years of refinement ensure
that an enzyme's binding sites are as molecule-specific and energy efficient
as possible. This translates to faster reactions and potentially less
expensive chiral synthesis, an advantage not easily matched by most synthetic
processes.
But there's one drawback. In order for an enzyme to produce a chiral
product, it must obtain electrons from a cofactor. Traditional cofactors,
such as the phosphate-and-sugar-equipped 1,4-NADH molecule used in Fish's
research, supply these electrons in the form of hydride ions. Unfortunately,
the complex 1,4-NADH molecule, like many cofactors, is unstable in air
and water and therefore has a shorter lifetime. Specifically, it is susceptible
to hydrolytic reactions that degrade the compound. This means that an
expensive 1,4-NADH cofactor may be required to make a less expensive pharmaceutical
product, meaning in some processes the cofactor is more expensive than
the product.
What's needed is a cofactor that's stable and fits seamlessly into complex
enzymatic reactions. Fish's team wondered if a biomimetic cofactor offered
the solution. In other words, replace the bulky, natural 1,4-NADH molecule
with a leaner, more stable mimic. Before setting their sights on chiral
synthesis, however, the team first had to ascertain whether this simpler
version works in a fundamental catalytic cycle that regenerates the needed
biomimetic cofactor, 1,4-NADH a process that essentially provides
the fuel for biocatalysis. In this catalytic cycle, the oxidized biomimetic
nicotinamide adenine dinucleotide (NAD+) cofactor grabs a hydride ion
from an organometallic rhodium catalyst. This hydride ion must always
attack the oxidized cofactor's carbon in the number four position, even
though the hydride ion has two other positions (two and six) to choose
from. This site-specific targeting, called regioselectivity, must occur
over and over again in order to sustain cofactor regeneration.
But does the simplified molecule exhibit the same regioselectivity as
its more complex cousin? To find out, Fish's team pared down the NAD+
molecule to its simplest form, replacing its sugar, phosphate and adenosine
groups with a simple benzyl group. They introduced this biomimetic version
into the catalytic cycle, and found that the regioselectivity remains
unchanged. The hydride ion attacks the simpler version's number four carbon
site to produce the reduced cofactor, 1,4-NADH, just as it does in the
naturally occurring compound.
"We can use the simplified NAD+ analog or the natural product; either
way it's the same," Fish says.
This solved the first piece of the puzzle. The hydride ion successfully
attacks the correct position on the stripped-down NAD+ molecule, a critical
intermediate step that enables the molecule to present the hydride ion
of the reduced cofactor to an enzyme.
Another, bigger roadblock loomed, however. Would the enzyme even recognize
the reduced biomimetic cofactor molecule? Enzymes are efficient because
they are engineered to interact only with specific molecules. This also
makes them finicky. Change a molecule even slightly, and the enzyme may
not accept it. Fish's team had to determine whether the stripped-down,
biomimetic 1,4-NADH is convincing enough for the enzyme's finely tuned
selection process.
They melded a catalytic cycle that regenerates the 1,4-NADH cofactor
with an enzymatic cycle in which a horse-liver alcohol dehydrogenase enzyme
accepts hydride ions from the 1,4-NADH to produce a chiral alcohol. Instead
of using a naturally occurring 1,4-NADH molecule that readily interacts
with the enzyme, they introduced their biomimetic version, stripped of
its sugar, phosphate, and adenosine groups. After running the reaction,
they determined that biocatalysis was successful: the biomimetic 1,4-NADH
cofactor offered its hydride ion to the enzyme, and the enzyme easily
accepted the hydride ion as if it were interacting with the natural compound.
This, in turn, precipitated an enzymatic process that resulted in a chiral
product. In addition, the reaction consistently produced a single chiral
product, which may suggest that biomimetic 1,4-NADH compounds can be used
in large-scale pharmaceutical and industrial processes.
Ultimately, the more such biomimetic cofactors are found to mesh with
a variety of enzymes to produce pharmaceutically valuable products, the
cheaper drug production might become, Fish says.
|
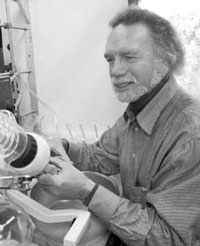 |
|
|
|
|
|
Richard H. Fish of Berkeley
Lab's Environmental Energy Technologies Division. |
|
"If you can use a cheaper biomimetic cofactor over and over again
without it decomposing, then you don't have to constantly reinvest in
replenishing the cofactor this saves money," Fish says. "This
also opens the door for researchers to determine whether other enzymes
can produce pharmaceutical products using biomimetic cofactors."
Berkeley Lab's John B. Kerr and former postdoctoral fellows H. Christine
Lo, Carmen Leiva, and Olivier Buriez also contributed to this research.
"Biomimetic NAD+ models for tandem cofactor regeneration, horse
liver alcohol dehydrogenase recognition of 1,4-NADH derivatives, and chiral
synthesis," by H. Christine Lo and Richard H. Fish, appeared in Angewandte
Chemie International Edition, 2002, 41, No 3.
|