A team of scientists at Lawrence Berkeley National Laboratory (Berkeley
Lab), using simultaneous ultrashort flashes of near-infrared and far-infrared
terahertz (trillion cycles per second) electromagnetic radiation, created
and observed electron-hole gases in semiconductor quantum wells as they
changed from electrical insulators to conductors and back to insulators
again.
The results demonstrate that semiconductor nanostructures, whose dimensions
are measured in billionths of a meter, are an ideal means for learning
more about Coulomb interactions in many-body systems -- those made up
of multiple interacting particles -- which lie at the heart of all our
technology in electronics and chemistry.
"We have developed a unique new way to study the dynamics of excitons
and unbound electron-hole pairs in semiconductors and got some surprising
results," says Daniel Chemla, director of Berkeley Lab's Advanced
Light Source and the physicist who led the research. "Our findings
open up a whole new set of questions about Coulomb interactions in many-body
systems, in particular in nanostructures."
|
|
 |
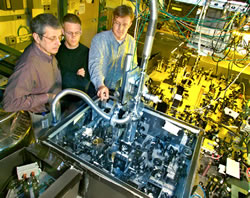 |
|
|
 |
Daniel Chemla, Mark Carnahan, and
Robert Kaindl examine their experimental set-up, incorporating more
than 100 mirrors. Each is computer controlled so that the entire assembly
can quickly be brought into precise alignment. |
|
|
Collaborating with Chemla on this experiment were Robert Kaindl and Marc
Carnahan, who are with Berkeley Lab's Materials Sciences Division, and
Daniel Hägele and Reinhold Lövenich, visiting researchers who
have since returned to Germany. The Berkeley team members are also affiliated
with the Physics Department of the University of California at Berkeley.
Results of this research, which was funded by the U.S. Department of Energy's
Office of Basic Energy Science, were published in the June 12, 2003 issue
of the journal Nature.
In a semiconductor crystal, absorption of visible light "photoexcites"
an electron into a higher energy space. The electron is moved into the
conduction band, up from the valence band where it is tightly bound to
the parent atom, and leaves behind a vacancy or "hole" that
acts like a positively charged particle. Electron and hole -- being oppositely
charged -- attract each other via the same Coulomb force that stabilizes
the shapes of atoms or molecules.
"If created by a sufficiently high-energy photon, this electron-hole
pair is unbound and can carry an electric current as its constituents
freely move around the crystal," explains Chemla. "Under the
right conditions, however, new quantum states called excitons can form,
with an electron and hole bound to each other. Owing to its local charge
neutrality, an exciton gas behaves as an insulator while a collection
of unbound electron-hole pairs form a conducting plasma. The transition
from electron-hole plasma to exciton gas is a typical metal-insulator
transition."
The internal energy levels of excitons are analogous to those of a hydrogen
atom, but the binding energy of excitons is about 1,000 times smaller,
as is the spacing of their energy levels. Until now, experimental investigations
of exciton dynamics have been indirect, as they probed excitons at photon
energies much higher than their level spacing.
Departing from this script, the Berkeley Lab researchers used near-IR
laser light to create the electron-hole pairs, then analyzed the results
with picosecond (trillionth of a second) pulses of far-infrared, terahertz
radiation. At a photon energy of about 4 meV (milli electron-volts), terahertz
radiation is the ideal light for observing the internal electromagnetic
transitions of excitons and unbound electron-hole gases.
"Working with semiconductors, experiments can be done in nanostructures
of exceptional purity and controlled growth," says Kaindl. "Conditions
including temperature, electron density, and magnetic fields can be varied
over many orders of magnitude, and the dimensionality can be custom tailored
so we can create Coulomb interactions of varying strengths."
|
|
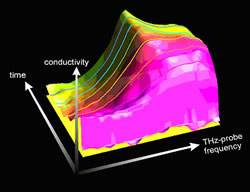 |
 |
|
|
Temporal snapshots of the correlated
motion between electrons and holes in a semiconductor nanostructure:
terahertz (THz) conductivity sharpens up on a picosecond time scale
as excitons take shape. |
|
|
|
Kaindl explains that "our experiment, in accessing the response
to terahertz radiation, gives us information about whether the constituents
in the electron-hole soup have paired up into an insulating state -- such
that their mutual strong attractive binding into excitons makes them virtually
immune to the small terahertz electric field -- or, alternatively, whether
they are still loosely associated in unbound states such that the external
terahertz field can induce an electric current. By using ultrashort pulses,
we can obtain a new view on the dynamic evolution of the many particle
system between these two extreme situations."
Through the combination of two-dimensional confinement in nanosized quantum
wells of undoped gallium arsenide, and tunable near-infrared photoexcitation
light from a titanium-doped sapphire laser, the Berkeley Lab researchers
were able to create, with a great degree of control, a plasma of electron
and hole pairs.
They then probed this plasma with picosecond pulses of terahertz radiation.
Generating both the pump and the probe light from the same source enabled
them to control very precisely the timing of events and access the transition
dynamics with excellent accuracy.
"Insulators and conductors live in two different worlds of experimental
apparatus, so usually scientists are looking at one or the other but not
both," said Chemla. "Ours is a unique experimental set-up and
allows us to study Coulomb interactions without the traditional need for
an electrical contact."
The experimental set-up, which was configured by Kaindl, features an
elaborate maze of about one hundred mirrors and other optics, precisely
positioned to deliver both the pump and the probe infrared pulses at a
desired length and frequency. Using picosecond pulse lengths, the researchers
were able to obtain a series of snapshot images showing the dynamic evolution
of the Coulomb interactions they generated.
Says Kaindl, "What we observed -- unexpectedly -- is a Coulomb correlation
enhancement at zero time delay after the excitation pulse has just created
a plasma of unbound electron-hole pairs. While the overall formation of
excitons takes place over a period of several hundred picoseconds, this
enhancement appears almost instantaneously and is related to the extremely
fast Coulomb interactions between the vast number of constituents of the
electron-hole plasma."
Adds Chemla, "In a normal metal, the density of charged particles
is so large that the Coulomb interaction is effectively screened. The
transient phase we observed seems to be something like a mixture of bound
and unbound electron-hole pairs. For now we are calling it 'strange metal,'
but we don't know what it really is yet."
While they expect their results will keep the theorists busy trying to
offer an explanation of this intermediate state during the formation of
excitons, the Berkeley researchers believe that the responses to terahertz
radiation they've demonstrated should pave the way for future studies
of Coulomb interactions in multibody systems that have remained elusive
to visible and near-infrared optics.
|