2004
RESEARCH PROJECTS
Integrative Studies (These Interdisciplinary projects integrate
research from more than one NABIR element)
|
PROJECT: |
Aqueous
Complexation Reactions Governing the Rate and Extent of Biogeochemical
U(VI) Reduction |
PRINCIPAL
INVESTIGATOR: |
Scott
Brooks |
Integrative
Studies |
Laboratory
research has shown that dissimilatory metal reducing bacteria (DMRB)
can effectively reduce oxidized uranium (U(VI)) to the sparingly
soluble U(IV) with the concomitant precipitation of UO2 phases. Despite the promise of
bioreduction as a remediation strategy, the factors thatenhance or inhibit the
rate and extent of biogeochemical U(VI) reduction under representative environmental
conditions are not well defined. Before effective bioimmobilization can be realized,
the factors governing contaminant reactivity in multicomponent systems must bebetter
understood. Only recently has the quantification of a few key interactions
been established. For example, we recently reported the inhibition of bacterial
U(VI) reduction by DMRB in the presence of environmentally realistic concentrations
of soluble calcium (Ca) (Brookset al., 2003). This finding has significant
implications for field applications of bioreduction because Ca2+ is a dominant
soluble and cation-exchangeable species in soils and aquifers. We propose
to identify and quantify the important biogeochemical reactions that alsoequilibrate
with the U-carbonate solution species and may inhibit or enhance U(VI) reduction. Initially,
cation exchange resins, with well-defined Ca2+ selectivities, will be employed
to establish the distribution of Ca-U-carbonate species in the presence of varying
amounts of cation-exchangeable forms of Ca2+; other potentially important competing
cations in the exchange equilibria (e.g., Mg2+, Sr2+) will be examined in later
phases of the proposed research.Concurrent with the measurement of the competing
equilibria among soluble and cation exchangeable phases, the reduction of the
major cation-U-carbonate species will be studied using both abiotic and microbial
agents. Our state-of-the-art measurement techniques (XAS,XRFS, EDX, TEM,
radioisotopes, ICPMS, and KPA) will be applied to quantify these soluble complexes
and precipitated phases. By understanding these important key equilibria,
more predictable and effective approaches can be established for in situ bioremediation
of Uunder realistic field conditions.
PROJECT: |
Reaction-Based
Reactive Transport Modeling Of Iron Reduction And Uranium Immobilization
At Area 2 Of The NABIR Field Research Center |
PRINCIPAL
INVESTIGATOR: |
William
Burgos |
Integrative
Studies |
The proposed
research is focused on developing mechanistic, phenomenological descriptions
of important reactions and mathematical formulations for modeling
reactions for the in situ immobilization of uranium promoted via
microbial iron(III) reduction. Our overall goal is to develop
and validate a robust mathematical model that can simulate all important
chemical reactions and physical processes associated with in situ U(IV)
immobilization. Experimental conditions will be designed to match
those in saturated zone sediments at Area 2 of the DOE NABIR Field
Research Center (FRC) in Oak Ridge, TN. This proposal is submitted
to the Integrative Studies element within NABIR as it examines
research topics related to both the Biogeochemistry and Biotransformation elements
and contains a significant mathematical modeling effort. Our
previous research has been funded through the Biotransformation element
(DE-FG02-98ER62691 and DE-FG02-01ER63180).
The research
will pursue three major objectives: (1) elucidate the mechanisms
of reduction of solid-associated U(VI) in Area 2 sediment at the
NABIR FRC; (2) evaluate the potential for long-term sustained U(IV)
reductive immobilization coupled to dissimilatory metal-reducing
bacterial (DMRB) activity in Area 2 sediments; (3) numerically simulate
the suite of hydrobiogeochemical processes occurring in experimental
systems so as to facilitate modeling of in situ U(IV) immobilization
at the field-scale. The proposed research is
based on the following five hypotheses: (1) the biological and chemical
reduction of sediment-associated U(VI) is fundamentally controlled
by its mineralogic and coordination environment; (2) the addition
of humic substances can stimulate the reduction of solid-associated
U(VI); (3) coupled Fe(III)/U(VI) reduction can be sustained in long-term
flow-through reactor experiments with hydrologic residence times
(week-to-month) comparable to those expected in pore domains likely
to be colonized by DMRB in Area 2 sediments; (4) modest levels of
nitrate input will not significantly inhibit coupled Fe(III)/U(VI)
reduction in the reworked fill of Area 2; and, (5) the kinetics and
thermodynamics of simultaneous biogeochemical reactions can be described
by a series of reaction-based kinetic and equilibrium formulations,
where rate formulations/parameter estimates derived from batch experiments
will be applicable to flow-through (semicontinuous culture and constructed
column) reactor experiments.
Both
experimental and model designs have been constructed to minimize complexity
of the conceptual systems, to build systems that can be validated based
on measurements readily made in the field, and to increase conceptual
complexity only when absolutely necessary. Based on these principles,
we have proposed integrated tasks to resolve our hypotheses and satisfy
our objectives. To address the first hypothesis we propose to
use surface complexation modeling and spectroscopic techniques (XAS,
LIFS) in a complementary fashion. U(VI) sorption experiments
will use Area 2 sediments and specimen minerals presumed to control
U(VI) sorption and reducibility in these sediments (specifically -
illite, goethite, and goethite-coated illite). The second hypothesis
will be tested using a series of biotic (Geobacter sulfurreducens)
and abiotic [excess Fe(II)] U(VI) reduction experiments with Area 2
sediments and specimen mineral assemblages in the presence/absence
of FRC humic substances in batch reactors, semicontinuous culture reactors
(SCRs), and constructed column reactors (CCRs). SCR experiments
will provide suspension samples over time for solid-phase analyses
(XAS, XPS, Mössbauer spectroscopy, ESEM, and wet chemistry). The
third hypothesis will be tested in long-term SCR and CCR experiments
containing Area 2 sediment inoculated with Geobacter sulfurreducens. Experimental
variables will include two hydrologic residence times (10 and 50 days)
and the presence/absence of FRC humic substances in the reactor feed
solution. The fourth hypothesis will be tested in long-term SCR
and CCR experiments with Geobacter metallireducens (capable
of both nitrate and metal reduction) and the presence/absence of 0.5
mM nitrate in the reactor feed solution. The fifth hypothesis
will be addressed via continued development and calibration/validation
of the BIOGEOCHEM – HYDROBIOGEOCHEM series of reaction-based
computer models. The proposed research will utilize two national
user facilities: the Advance Photon Source (APS) at Argonne National
Laboratory, and the W.R. Wiley Environmental Molecular Sciences Laboratory
(EMSL) at PNNL. Our graduate students will be hosted at EMSL
by PNNL collaborators for 8-week periods of intensive summer research
during each year of the project.
PROJECT: |
Integrated
Nucleic Acid System for In-Field Monitoring of Microbial Community
Dynamics and Metabolic Activity |
PRINCIPAL
INVESTIGATOR: |
Darrell
Chandler |
Integrative
Studies |
Molecular
analysis of subsurface microbial communities requires some combination
of sample collection, concentration, cell lysis, nucleic acid purification,
PCR amplification and specific detection in order to address fundamental
questions of microbial community dynamics, activity and function in
the environment. As a result of our prior NABIR
grant, we have now developed a suite of integrated microparticle chemistries,
Fe- and SO4-reducer phylogenetic probes and suspension arrays, and combined
sample purification/bead array fluidic devices for the automated and
direct analysis of 16S rRNA and microbial community dynamics in environmental
samples. However, changes in microbial community composition and/or
abundance are still insufficient to detect or make conclusions regarding
specific microbial activity. Thus, fieldable methods for
the direct analysis of functional genes and messenger RNA (mRNA) in the
environment are still required. The objective of the proposed work
is therefore twofold. First, we seek to verify the 16S rRNA methods,
Fe- and SO4-reducer array and instrumentation on sediment and groundwater
samples taken from the Schiebe/Roden FRC site, and continue to expand
the probe suite for microbial community dynamics as new sequences are
obtained from DOE-relevant sites. Second, we propose to address
the fundamental molecular biology and analytical chemistry associated
with the direct analysis of functional genes and mRNA (hence, microbial
activity) in environmental samples. These studies will investigate
the behavior of oligonucleotide and cDNA capture and detection probes
for direct mRNA purification and detection on microparticle surfaces; “tunable
surface” chemistries to increase mRNA capture and detection efficiency;
new mRNA reporter and detection chemistries required for the development
of in-field monitoring methods and devices; and fluidic strategies for
integrating complex biochemistry for direct detection of mRNA in sediments
and groundwater, without using the polymerase chain reaction (PCR).
PROJECT: |
Characterizing
the Catalytic Potential of Deinococcus, Arthrobacter and
other Robust Bacteria in Contaminated Subsurface Environments of
the Hanford Site |
PRINCIPAL
INVESTIGATOR: |
Michael
Daly |
Integrative
Studies |
Immense volumes of soil and groundwater at numerous U. S. Department
of Energy (DOE) sites have low levels of widespread contamination
that include mixtures of heavy metals (e.g., Hg & Cr)
and radionuclides (e.g., U & Tc), as well as chlorinated
hydrocarbons. The remediation of such contaminated sites constitutes
an immediate and complex waste management challenge for DOE, particularly
in light of the costliness and limited efficacy of current physical
and chemical strategies for mixed wastes. In situ bioremediation
via natural microbial processes (e.g., metal reduction)
remains a potent, potentially cost-effective approach to the reductive
immobilization or detoxification of environmental contaminants.
Several key findings for bacteria belonging to the families Deinococcaceae and Arthrobacteriaceae support
their suitability as subjects for the Integrative Study element of
NABIR, combining the elements Biomolecular Sciences and Engineering,
and Community Dynamics and Microbial Ecology. We have recently isolated
several distinct species of Deinococcus and Arthrobacter from
pristine and contaminated soils of DOE’s Hanford Site in south-central
Washington state. Generally, Deinococcus bacteria are exceptionally
robust, not only surviving exposure to radiation, but also to other
DNA damaging conditions typical of DOE environments. The ability
to reduce a variety of toxic metals also appears to be a characteristic
shared by these organisms, and we have shown that D. radiodurans and D.
geothermalis are proficient at reducing U(VI), Tc(VII), (in
the presence of an electron shuttle) and Cr(VI), and have engineered
both species for Hg(II) reduction. Recent experimental advances in
the genetic management of D. radiodurans and D. geothermalis will
facilitate our efforts to characterize the metal-reducing mechanisms
of both organisms. These advances include our comprehensive analysis
of the D. radiodurans genome, and for D. geothermalis,
the development of a system for genetic transformation and ongoing
genome sequencing, annotation, and analysis of this organism. Arthrobacter species
are capable of free-living growth in many extreme environments, such
as under chronic radiation. Arthrobacter spp. have also
recently been reported to efficiently resist and reduce high concentrations
of hexavalent chromium. These and other cultured isolates from the
Hanford Site will be examined for their metal-reducing capabilities
as outlined below.
The
four specific aims of this proposal are: 1) Characterizing the genetic
basis of metal reduction in D. radiodurans and D. geothermalis,
and novel deinococcal isolates from the Hanford Site; 2) Characterizing
the microbial ecology of contaminated waste sites and metal-reducing
potential of these communities; 3) Defining environmental factors that
govern in situ growth, environmental robustness, metabolism,
and Cr(VI)-reduction of Deinococcus and Arthrobacter spp.;
and 4) Characterizing the genetic basis of metal reduction in Arthrobacter
aurescens (TC1) and novel Arthrobacter isolates from
the Hanford Site.
PROJECT: |
Biogeochemical
Mechanisms Controlling Reduced Radionuclide Particle Properties
and Stability |
PRINCIPAL
INVESTIGATOR: |
James
Fredrickson |
Integrative
Studies |
Uranium
and technetium, common subsurface contaminants on DOE sites, are
problematic because they exist as highly soluble and mobile ions,
U as U(VI) carbonate complexes and Tc as (Tc(VII)O4-), in oxidized
groundwaters. Laboratory research has demonstrated that dissimilatory
metal-reducing bacteria (DMRB) can effectively reduce U(VI) and Tc(VII)
to insoluble UO2 and TcO2 phases. Bioreductive immobilization
of these contaminants offers considerable promise for in situ remediation
of contaminated DOE sites. A major focus of the NABIR program
is on “understanding the role of microorganisms in long-term immobilization
of contaminants in place, and the potential for their remobilization”. Considerable
emphasis has been placed on defining the specific biological and biogeochemical
mechanisms of contaminant reduction within the NABIR program yet relatively
little is know regarding the biological factors controlling the form
and behavior of the bioreduced contaminants.
Our previous NABIR research findings demonstrated that UO2 nanoparticles
are present in the cell periplasm of metal-reducing Shewanella sp.
and that localization of UO2 to this compartment protects U(IV) against
oxidation by Mn(III,IV) oxides. More recently, we have established
that UO2 and TcO2 particles of relatively uniform nm-size are transported
from the periplasm via a protein secretion pathway that is common to
many Gram-negative bacteria. This is a rather remarkable finding
and the first report we are aware of describing the transport of a solid
phase across the outer membrane of bacteria. This process has important
implications for the long-term immobilization of contaminants and potential
for their remobilization. Once nanoparticles are “secreted” from
cells they may be subject to a variety of processes including oxidation
and transport. This research will focus on defining the mechanisms
by which Gram-negative metal-reducing bacteria export contaminant nanoparticles
from the periplasm and on the subsequent biogeochemical behavior of the
externalized nanoparticles.
PROJECT: |
Biogeochemical
Cycling and Environmental Stability of Pu Relevant to
Long-Term Stewardship of DOE Sites |
PRINCIPAL
INVESTIGATOR: |
Bruce
Honeyman |
Integrative
Studies |
Plutonium
contamination is widespread in surface soils and subsurface sediments
throughout the DOE complex. Until the last decade or
so, Pu was generally considered to be relatively immobile in the terrestrial
environment, with the exception of transport via aeolian and erosional
mechanisms. More recently, however, the transport of colloidal
forms of Pu has been invoked as providing a mobilization pathway in
low intensity stream environments and the subsurface.
Central
to understanding the environmental behavior of Pu in vadose- and
saturated-zones, as well as waste streams, is the contribution of
microbial communities to Pu speciation. This
proposed research would address the principal mechanisms by which naturally
occurring microbial communities regulate transformations in Pu chemical
speciation; such changes may lead to either enhanced Pu immobilization
or its release from immobile phases and subsequent transport.
The overall
objective of this proposed research is to understand the biogeochemical
cycling of Pu in environments of interest to long-term DOE stewardship
issues. Central to Pu
cycling (transport initiation —> immobilization)
is the role of microorganisms. The hypothesis underlying
this proposal is that microbial activity is the causative agent
in initiating the mobilization of Pu in near-surface environments:
through the transformation of Pu associated with solid phases,
production of extracellular polymeric substances (EPS) carrier
phases, and the creation of microenvironments. Also,
microbial processes are central to the immobilization of Pu species,
through the metabolism of organically complexed Pu species and
Pu associated with extracellular carrier phases and the creation
of environments favorable for Pu transport retardation.
The
following figure illustrates the information flow in this multi-institution
project.
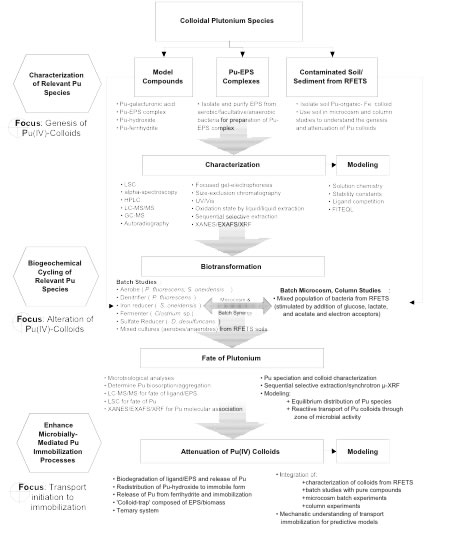
Experimental
approach and information flow. This work is an
integrated program between the three participating institutions. The
components of study include the use of model compounds, environmental
materials and bacterial exudates produced under controlled laboratory
conditions.
PROJECT: |
Stabilization
of Plutonium in Subsurface Environments via Microbial Reduction
and Biofilm Formation |
PRINCIPAL
INVESTIGATOR: |
Mary
Neu |
Integrative
Studies |
Subsurface actinide contaminants at DOE sites that have complicated
hydrogeology and redox environments are affected by direct and indirect
microbially-mediated processes. The influence of these processes is
particularly difficult to predict for plutonium, due to its complicated
redox behavior and rich chemistry. We propose to investigate how key
reactions, which are known to affect major redox-active transition
metals, such as Fe and Mn, can affect Pu speciation and environmental
mobility. The overarching goal of the proposed research is to understand
and optimize mechanisms for in situ immobilization of Pu species
by naturally-occurring bacteria, beginning with an investigation of
a) bacterial accumulation and immobilization of Pu species by biofilm
formation and b) bacterial mineralization and immobilization via direct
enzymatic and indirect biogeochemical reduction of Pu species by metal
reducing bacteria. A combination of aqueous chemical, radioanalytical,
spectroscopic and microscopic analyses will be used to characterize
Pu speciation and solution/solid phase distributions. Biotransformation
and biogeochemical research results will fill significant gaps in the
scientific basis for monitored natural attenuation and in situ stabilization
of widespread and problematic Pu contamination, as well as provide
immediately useful data to modeling and risk assessment efforts.
PROJECT: |
An
Integrated Assessment of Geochemical and Community Structure Determinants
of Metal Reduction Rates in Subsurface Sediments |
PRINCIPAL
INVESTIGATOR: |
Anthony
Palumbo |
Integrative
Studies |
Significant advances have been made in the ability to measure the composition
of indigenous subsurface microbial communities. However, fundamental questions
still persist concerning the interactive effects of geochemistry and community
structure on metal reduction rates in the subsurface. Many microorganisms can
change the subsurface geochemical conditions (e.g., cause a drop in redox) so
metal reduction becomes an energetically favored reaction. Some microbes can
directly catalyze the necessary reactions so that metal reduction occurs at a
more rapid rate than without microbial activity. Many microorganisms can accomplish
the first role but many fewer can accomplish the second. Physical and geochemical
factors such as mass transport, oxygen level, and nitrate concentration will
likely dominate the rate of microbial change in the redox potential. Thus, it
is possible that the importance of community structure at this stage of metal
reduction may be minimal. However, the effect of community composition on the
rate of metal reduction may be important. We will use controlled laboratory experiment
with sediments and groundwater from the NABIR Field Research Center (FRC) to
compare the effects of manipulations designed to influence community structure
(differences in electron donors) to those designed to influence geochemistry
(presences of humics as electron shuttles) on uranium reduction. Also, the effect
of carbon:phosphate ratios on community structure and uranium reduction rates
will be examined in the context of resource-ratio theory to help predict the
nutrient supply rates and ratios that maximize uranium reduction at the FRC site.
In the final stage we will extend the research to other sites where toxic metals
and radionuclides are problematic.
[Back
to Award Recipients Page]
|