Reprinted from the Fall/Winter 1992
Research Review Magazine
Of all the tools and instruments of science, there is none so grand or
enduring as the telescope. Looking up at the heavens, a telescope brings
us images of the past that say much about the present and provide clues
about the future. Some telescopes "see" radio waves, some see
x-rays, and some see the heat given off by celestial objects; but the
bread and butter tools of modern astronomy remain the optical telescopes,
those that see visible light--the principal radiation of the stars.
This past spring, construction was completed on the largest optical
telescope in the world, the W. M. Keck Telescope on the island of Hawaii.
With a light-gathering mirror that measures 10 meters (400 inches) in
diameter, the Keck Telescope will essentially double the observation range
of any existing telescope, bringing into view objects more than 10 billion
light years away (one light year is equal to about 6 trillion miles).
Built and operated by the California Association for Research in
Astronomy (CARA), a partnership of the University of California (UC) and
the California Institute of Technology (Caltech), the Keck Telescope
represents the first major departure in fundamental optical telescope
design since the days of Sir Isaac Newton. This design breakthrough, which
has been reproduced in a twin 10-meter telescope adjacent to the first,
was born and nourished at LBL.
In 1977, UC formed a five-member committee to come up with a design for
a proposed ground-based optical telescope whose reflecting mirror would be
double the size of the Hale Telescope at Mount Palomar, the 5-meter
(200-inch) behemoth that had been the nation's biggest telescope since
1948. The committee included representatives from each of the UC campuses
with major astronomy programs--Berkeley, Santa Cruz, Los Angeles, and San
Diego--plus an experimentalist with LBL's Physics Division, whose training
and background had been in particle physics, but who had become involved
in studies of pulsars and neutron stars. This astrophysicist was Jerry
Nelson.
Initially, when the UC committee met, the idea was to design
essentially a scaled-up version of the Hale Telescope, a telescope with a
monolithic reflecting mirror. This approach presented a number of
problems. A reflecting mirror with a 10- meter diameter would require an
elaborately complex structural support system to keep it from collapsing
under its own enormous weight. Also, the larger a mirror's surface, the
thicker it must be in order to withstand gravitational effects that could
alter its shape.
As the size swells, the cost of the mirror becomes exorbitant. It was
estimated that the cost of a 10-meter telescope, using a single reflecting
mirror, could top a billion dollars. Everyone agreed that the prospects
for funding a billion-dollar telescope were slim.
Nelson had a better idea.
Instead of a single, gigantic reflecting mirror, Nelson proposed
constructing a parabolic or bowl-shaped reflecting surface out of many
thin mirror segments. He argued that if the technology of a segmented
mirror could be mastered, there would be no inherent limit to the size of
a reflecting surface.
Said Nelson in an interview at the time, "The Hale Telescope was
very innovative for its day, but in terms of advancing the state of the
art--or at least pushing the available technology to its limits--it's been
downhill ever since for optical telescopes. It is time for a forward step,
not just making improvements in an old design."
The UC committee continued to favor the monolithic mirror approach but
was intrigued enough to invite Nelson to develop his idea. He spent the
next two years working at LBL with a number of researchers, most
prominently, physicist Terry Mast and engineer George Gabor. In 1979, he
presented their segmented mirror approach to the committee, and it was
chosen over several other concepts.
Specifically, the design that Nelson and his colleagues settled on
called for a mosaic of 36 hexagonal mirror segments arranged in the form
of a honeycomb and kept in perfect alignment by a computer-operated active
control system. Each mirror segment would be 1.8 meters wide, 7.5
centimeters (about 3 inches) thick, and weigh about half a ton. Taken as a
whole, this segmented reflecting mirror was expected to be about the same
weight as the Hale Telescope's mirror even though it would be quadruple
the size in surface area.
 |
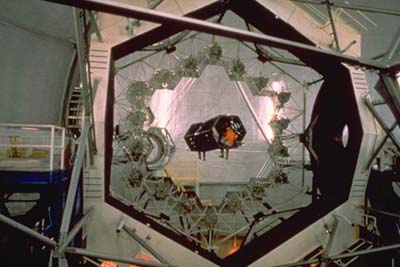
THE UNIQUE MOSAIC DESIGN OF THE KECK PRIMARY MIRROR CONSISTS
OF 36 HEXAGONAL SEGMENTS. THESE SEGMENTS ARE ALIGNED TWICE A
SECOND TO AN ACCURACY OF FOUR NANOMETERS -- OR 1,000 TIMES THINNER
THAN A HUMAN HAIR -- THUS FORMING A VIRTUALLY CONTINUOUS PIECE OF
GLASS.
Photo courtesy of the W.M. Keck Observatory
|
Nelson and his LBL colleagues faced two major hurdles: designing a
means of keeping the 36 segments perfectly aligned, and finding a way of
polishing the segments so that together they would function as a single,
giant, parabolic surface.
The surface of the reflecting mirror of any large telescope is
continually subjected to stress from such factors as atmospheric
temperature changes, shifting winds, or changes in the mirror's position
as it scans the sky. Nelson knew that such stresses would readily disturb
the alignment of his proposed mirror segments, so developing a system that
could compensate for these stresses was essential.
The alignment system Nelson, Mast, and Gabor finally devised consisted
of 168 electronic sensors mounted on the edges of the hexagonal mirror
segments, and 108 individual, motor-driven adjusting mechanisms, called
"position actuators," which are connected to the back of the
segments at the rate of three actuators per segment. The sensors on each
segment continually compare the height difference between the segment and
its neighbors. If a segment moves with respect to its neighbors even as
little as a millionth of an inch--a thousand times thinner than a human
hair--this information is instantly relayed to a computer. The computer
collects data from all 168 sensors, calculates what adjustments are
necessary to bring all of the segments back into alignment, then directs
the actuators to make these adjustments. By the time one batch of
correcting movements has been completed, a new reading has been taken and
the process begins again. Alignment of the Keck's entire array of mirror
segments occurs twice every second.
In addition to this "active" mirror support system, a team of
LBL researchers designed a "passive" support system. The passive
support consists of a thin, stainless-steel "flex disk" attached
to the back of each segment and three "whiffletrees" with
articulating arms that "float" the segment at 36 evenly
distributed points, as if there were no gravity. Working in tandem, the
flex disk and whiffletrees resist side-to-side motions but permit the up
and down and tilting movements needed to align the segments.
Nelson credits much of the solution to the mirror-polishing problem to
Jacob Lubliner, a professor of engineering with UC Berkeley. Adapting a
technique first used by the German telescope designer Bernhard Schmidt to
fashion a combination reflection-refraction mirror into a huge camera,
Nelson, working with Mast and Lubliner, developed a technique called
"stressed mirror polishing."
In this technique, forces and torques are selectively applied to the
edges of a mirror blank in order to warp the blank to a desired degree of
distortion. A simple sphere is then polished into the distorted blank, and
the forces and torques are removed. If the correct forces were applied,
the spherical surface will elastically relax into the desired shape.
Explained Nelson, "Polishing a segment of a parabolic curve is
practically impossible, the mirror segment would have to be the shape of a
potato chip. But polishing a segment of a sphere is quite routine."
Knowing the final shape of each mirror segment they wanted, it was a
simple matter for Nelson and his colleagues to determine the amount of
distortion they needed. Solving the equations governing elasticity for the
glassy ceramic material they used as a mirror blank yielded the forces
needed to achieve this distortion.
Once a mirror blank was polished, it could be cut into a hexagon.
Nelson and his colleagues later learned that the cutting operation
slightly distorted a mirror's parabolic pattern. However, the attachment
of a harness, consisting of 30 metal leaf springs, to the back of the
mirror could impose forces on the mirror that would bend it back into the
required shape.
In 1984, a technical demonstration of the alignment and control system
was held at LBL using a full-size mirror segment and a prototype sensor
and actuator. The demonstration proved that the system worked.
During this period, there was also intense activity on the structural
design of the telescope, led by UC Berkeley's Steve Medwadowski, after a
preliminary design effort led by Lubliner. Techniques for moving the
telescope and sensing its position were pursued by Jack Osborne of UC's
Lick Observatory. Bob Weitzmann of Berkeley's Space Sciences Laboratory
made fundamental contributions to the passive support system and its
attachment to the telescope structure. After an intensive review of all of
this work, astronomers from California Institute of Technology agreed to
join UC in the project. The search for funding began in earnest.
The donor that was found was the W.M. Keck Foundation of Los Angeles.
Named for William M. Keck, the founder of the Superior Oil Company, this
foundation is among the nation's largest charitable organizations. At the
beginning of January 1985, the foundation announced it would grant $70
million to Caltech to build the telescope. Caltech and UC officially
formed CARA. Under the agreement, Caltech would provide the money to build
the telescope (approximately $94.5 million) and UC would provide the money
to operate it for roughly 25 years.
On September 12, 1985, ground was broken to begin construction on a
cinder cone atop an inactive volcano called Mauna Kea, widely acknowledged
as the best site on the face of the Earth for a telescope. At an elevation
of 13,796 feet, the peak of Mauna Kea is above 40 percent of the
atmosphere. The thin air up there is dry, usually cloudless, and far
removed from the polluting chemicals and urban lights that hamper other
observatories. Mauna Kea is also surrounded by a thermal blanket, the
Pacific Ocean, which greatly reduces the air turbulence that can disrupt
visible light observations.
When CARA's construction of the Keck Telescope began, Nelson was named
the project scientist. Named as project manager was Jerry Smith of the Jet
Propulsion Laboratory, who had previously managed the construction of
NASA's Infrared Telescope Facility on Mauna Kea and who also oversaw the
international collaborative effort to develop the Infrared Astronomical
Satellite.
LBL's Engineering Division continued to play a vital role in the
project. Taking over project manager duties for LBL was Engineering's Andy
DuBois. Dick Jared was placed in charge of work on the active support
system, and Bob Fulton was named to lead the passive support system work.
Steve Lundgren and Bob Minor also played prominent roles. (Jared and Minor
will be heading up LBL's contributions to the construction of the twin
telescope, called Keck II. LBL will be receiving some $750,000 over the
next four years to serve in a consulting role in the fabrication of the
Keck II active support system.)
By the summer of 1987, groundwork for the Keck telescope had been
completed, the concrete foundation had been poured, and construction had
begun on the dome. Experience has taught astronomers that even small
sources of heat within a dome can distort or seriously degrade the images
captured by a telescope's primary mirror. Consequently, CARA went to great
lengths to remove from the Keck's dome all sources of heat. The dome's
interior walls are insulated and covered with a paint chosen for thermal
properties that minimize internal heating during the day. During the day,
the interior is air-conditioned, and huge fans can replace all of the air
inside the dome every five minutes if necessary. This keeps the indoor and
outdoor temperatures nearly identical and ensures that the quality of the
mirror's image is limited only by the natural properties of the
atmosphere. The thermal design of the dome was studied and optimized by
Bill Carroll of LBL's Energy and Environment Division.
A significant savings in construction costs and materials was achieved
because the Keck's dome could be made significantly smaller than the dome
of the Hale Telescope. The dome's compact size--it measures 30 meters high
and 36 meters wide--was possible because of the extremely short focus of
the Keck's segmented mirror and because the Keck uses what is called an
alt-azimuth mounting rather than a conventional and considerably bulkier
equatorial mounting.
While the dome was being erected, the Keck's tube structure was being
assembled in Spain and tested for flexure. This eight- story structure was
designed to provide maximum strength and stiffness with a minimum amount
of steel. Thanks to its unique design, and to the fact that the mirror
segments are so much thinner than a 10-meter monolithic mirror would have
to be, the overall weight of the Keck Telescope is 298 tons, about half
that of the Hale Telescope.
In 1989, workers began installing the Keck telescope's steel tube
structure inside the dome. The space-frame mirror cell that holds the
mirror, which consists of more than 1100 individual working pieces, was
added next; and on October 12, 1990, the first mirror segment was put into
place. Two months later, on December 4, with nine of its mirror segments
in place, the telescope captured its first light.
CARA scientists released a dramatic photo of NGC 1232, a pinwheel
galaxy some 65 million light years away. Even with only a fourth of its
primary mirror installed, the Keck telescope already matched the
light-collecting power of the Hale Telescope.
Said an exuberant Nelson during the press conference at which the photo
was released, "We are jumping up and down over the sharpness of the
image."
At the same conference, Lick Observatory director Robert Kraft said,
"The image is pretty spectacular. At this stage of the game, I don't
know of any telescope in modern times that worked properly right off the
bat like this."
The W.M. Keck Observatory was officially dedicated on November 7, 1991,
with a ceremony that included in addition to the usual speeches, a chanter
and priest who offered traditional Hawaiian blessings of the telescope and
the people who will work with it, and a traditional Hawaiian ground
blessing of the site for the Keck II. On April 14, 1992, the final mirror
segment was lowered into the cell, and the telescope was complete.
"I'm ecstatic," was Nelson's official comment.
New telescopes generally require a year or more of shakedown time to
become fully operational. During this year, Nelson, working with Smith and
other CARA members, will be testing the telescope and fine-tuning its
optics to obtain the best images possible.
One of the key tests that has already been passed was whether or not
the warping harnesses would work as they were supposed to and correct the
shape of each mirror segment to within one-millionth of an inch.
"With these corrections, we hope to get a resolution of 1/2 second
of arc (astronomers measure resolution in angles, one arc second is
1/3600ths of a degree) or better when the atmosphere is calm," said
Nelson. "This is roughly equivalent to being able to distinguish a
car's headlights as two separate objects from a distance of 500 miles, and
is about twice as good as the Keck's first images."
To capture its images, the Keck will use either a Cassegrain or a
Nasmyth focus. In the Cassegrain focus, light from an object being viewed
will be gathered by the mosaic of mirror segments and reflected back
toward the prime focal point. Before reaching the prime focal point,
however, the light will be reflected once again by a small convex
secondary mirror, held in a steel frame about 15 meters above the primary
mirror, back through an opening in the center of the primary mirror. In
the Nasmyth focus, an oblong tertiary mirror is positioned in this opening
and it reflects the light from the secondary mirror to foci located on
either side of the telescope.
There are two secondary mirrors that can be used on the Keck
telescope--one for optical studies and one for infrared studies. One of
the first telescopes designed for both visible light and infrared viewing,
the Keck's infrared capabilities are expected to be far superior to those
of any other telescope now in operation. It will make infrared
measurements 40 times faster-- meaning it can see much fainter sources of
radiation--and produce infrared photos three times sharper than any
telescope before.
The dry air above Mauna Kea is a further boost to the Keck Telescope's
infrared capabilities. These capabilities will enable the Keck telescope
to penetrate areas of space too clouded by dust and gas for clear,
visible-light sightings. Infrared viewing is particularly useful for
finding protostars--stars still in the formation process--that radiate a
great deal of heat but are enshrouded in an opaque cocoon of star stuff
that makes them appear as smudges to an optical telescope.
Whether the telescope is operating as an optical or an infrared
instrument, rarely will there be an astronomer peering through an eyepiece
at the Keck's images. Instead, images will be recorded by
computer-controlled cameras. This is because, to the human eye, a dim
object will appear dim no matter how long it is stared at, whereas
photographic film, or other types of detectors, exposed to a dim light for
a long enough time will produce a bright image. Where the telescope looks
will also be computer-controlled. A telephone line linked to the computer
allows telescope-pointing instructions to be phoned in.
UC and Caltech astronomers will each be allotted 45 percent of the
telescope's observation time, and astronomers with the University of
Hawaii will get the remaining time. Some time from each group, however,
will be granted to astronomers from other institutions. One idea that will
be tested is for astronomers to send their observation requests to a
permanent staff at the observatory, which will collect the data and send
it back to the requesters. Peter Gillingham, the former officer-in-charge
of the Anglo-Australian Observatory in New South Wales, was recently named
the first operations director of the W. M. Keck Observatory.
With its gigantic primary mirror and its optical and infrared secondary
mirrors, the Keck telescope will be a time machine, enabling astronomers
to observe the history of our universe as it unfolds. All optical
telescopes look back through time, seeing an object the way it appeared
when its light or reflected light originated, just as when we look at
someone on the other side of a room, we actually see them as they looked a
hundred millionth of a second ago. At such short distances, this time
lapse is, of course, insignificant, but it becomes very significant when
looking at stars.
For example, some 10 billion light years away from Earth are
quasi-stellar objects or "quasars," the most luminous objects in
the universe, putting out hundreds of times the energy of the entire Milky
Way. Many astronomers believe that quasars are the brilliant cores of
galaxies as they were at the beginning of creation. In providing
unprecedented views of quasars, the Keck telescope will show astronomers
how the universe appeared when time began, shortly after the Big Bang that
most scientists believe started the transformation of radiation into
matter.
The Keck telescope will also provide a look at the past, present, and
future of stars, including our own sun, through detailed spectral analyses
of hot, young, blue stars, middle-aged yellows, old and dying red giants,
and the final death throes of the white dwarfs. In such an analysis, a
prism is used to break down the ordinary white light of a star into a
spectrum whose rainbow of colors will be slashed with narrow dark or
bright lines. These lines are the spectral signatures of a particular atom
or molecule, and from them astronomers can determine a star's chemical
composition, its distance from Earth, its age, surface temperature, and
gravity, how it is moving through space, and even whether or not it is
rotating. Such spectral analysis requires collecting a great many photons,
and for that there will be none finer than the Keck telescope with its
brute aperture.
The brute aperture of the Keck Telescope will also be brought to bear
on one of the greatest searches of all astronomy, the search for stars
with planetary systems like that of our sun. In 1996, when the Keck II is
scheduled to be completed, the twin telescopes will operate as an
interferometer. Interferometry is a technique whereby the light from the
primary mirrors of two or more telescopes is converged to form a single
image. This not only doubles, or more, the light-collecting power, it
greatly enhances resolution, from 1/2 an arc second, which is considered
the limit for the best telescopes today, to only a few thousandths of an
arc second. Operating as an interferometer, the Keck and Keck II are
expected to have enough resolution to search for Jupiter-sized planets
around the 100 nearest stars.
Before construction of the Keck telescope began, Nelson was asked what
answers to cosmological questions he thought his brainchild would provide.
In response, he mused that it might be even more exciting to ponder the
questions that the Keck might raise: "With this telescope, we might
see something completely unexpected out there, and that's the real reason
you build a telescope--to see the things you have not anticipated."
Nelson's sentiments echoed those of Galileo who, in describing the
wondrous sights he'd viewed with his telescope, said, "I discovered
in the heavens many things that had not been seen before our age."

Sidebar: The Evolution of the Telescope
The telescope was invented in 1608 by a Dutch optician named Hans
Lippershey. It was introduced to astronomy in 1609 by the great Italian
scientist Galileo Galilei, who became the first man to see the craters of
the moon, and who went on to discover sunspots, the four large moons of
Jupiter, and the rings of Saturn. Galileo's telescope was similar to a
pair of opera glasses in that it used an arrangement of glass lenses to
magnify objects. This arrangement provided limited magnification--up to 30
times for Galileo--and a narrow field of view; Galileo could see no more
than a quarter of the moon's face without repositioning his telescope.
In 1704, Newton announced a new concept in telescope design whereby
instead of glass lenses, a curved mirror was used to gather in light and
reflect it back to a point of focus. This reflecting mirror acts like a
light-collecting bucket: the bigger the bucket, the more light it can
collect. The reflector telescope that Newton designed opened the door to
magnifying objects millions of times--far beyond what could ever be
obtained with a lens.
Over the next two centuries, there were modifications to the method of
focusing, but Newton's fundamental principle of using a single curved
mirror to gather in light remained the same. The major change that took
place was the growth in the size of the reflecting mirror, from the 6-inch
mirror used by Newton to the 6-meter (236 inches in diameter) mirror of
the Special Astrophysical Observatory in Russia, which opened in 1974.
The idea of a segmented mirror dated back to the 19th century, but
experiments with it had been few and small, and many astronomers doubted
its viability. It remained for the Keck Telescope to push the technology
forward and bring into reality this innovative design.
|