|
 |
BERKELEY, CA. -- Its the surface of a
solid that contacts liquids, gases, and other solids, so its no surprise that a lot
of interesting chemistry happens on the surface of things. Scanning tunneling microscopy
(STM) is one of the best ways to get a close look: the tip of a tiny
electrode moves over a sample and, by measuring the current due to electrons tunneling
through the gap between the electrode and the sample, creates a picture of the surface
virtually atom by atom.
 |
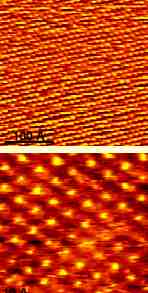 LOW-RESOLUTION
(ABOVE) AND HIGH-RESOLUTION IMAGES (BELOW) OF CLEAN PLATINUM EXPOSED TO A MIX OF CARBON
MONOXIDE AND OXYGEN. THE IMAGES SHOW AN ORDERED STRUCTURE OF CARBON-MONOXIDE
MOLECULES FORMING A MOIRÉ PATTERN DUE TO THE MISMATCH WITH THE PLATINUM LATTICE, AFTER
THE SAMPLE WAS HEATED TO 456 K. |
Traditionally, STM is done in high vacuum; some chemical reactions, however, including
important catalytic reactions, occur only under pressure. There is no dependable way to
extrapolate from high-vacuum STM experiments to high pressure research on catalysis.
"A while ago the idea of uniting these two communities -- the high-vacuum STM
community and the people who investigate catalysis under high pressure and temperature --
just jumped out at me," says Miquel Salmeron of Berkeley Labs Materials
Sciences Division, who in 1993 built the first scanning tunneling microscope capable of
working with samples under heat and pressure.
"Some kinds of atomic-scale studies can only be done in vacuum, because many of
the techniques used in them -- low-energy electron diffraction or electron microscopy, for
example -- are based on electrons traveling out of the sample, and gas molecules diffuse
these electrons," Salmeron explains. "With STM, however, although high vacuum is
useful to keep the sample and the microscopes probe tip from reacting with air or
other gases, theres no intrinsic reason it has to be done in vacuum."
Recently Salmeron and his colleagues, including Gabor Samorjai, John Jensen, and Keith
Rider, have used the new technique to discover unsuspected features of catalytic reactions
on the surface of platinum at the atomic scale.
A catalyst is a material or chemical that speeds up reactions without itself undergoing
permanent change. In the catalytic converters of automobiles, platinum is used to promote
the conversion of exhaust gases -- carbon monoxide and unburned hydrocarbons -- to carbon
dioxide and water. What happens when carbon monoxide adheres to an exposed platinum
surface?
Salmeron and his colleagues showed that the answer critically depends on pressure and
temperature. Despite what some researchers had argued, the molecular structure of carbon
monoxide adsorbed on platinum at ambient temperature and high pressure is fundamentally
different from the structures seen in vacuum at an extremely cold temperature, even when
similar amounts cover the surface.
"In a cold vacuum," says Salmeron, "carbon monoxide molecules form
ordered structures that are different and may not represent equilibrium structures but
rather metastable ones, frozen in place. Under heat and pressure, however, the carbon
monoxide covers the surface uniformly. This layer is not only in equilibrium with the gas;
due to the much higher temperature, it also has had time to find its true spatial
equilibrium arrangement."
A close-packed hexagonal lattice of carbon monoxide overlies the platinum substrate
atoms, in the ratio of three carbon monoxide molecules to approximately four platinum
atoms, forming a striking hexagonal moiré pattern. "Moiré patterns result when two
regular arrays overlap and give rise to a third periodicity," Salmeron explains.
It was Salmerons inspiration to mount a scanning tunneling microscope inside an
air-tight chamber immediately adjacent to a traditional high-vacuum chamber. The platinum
crystal is first prepared in the high-vacuum chamber; samples can also be characterized
here using standard techniques such as low-energy electron diffraction and Auger
spectroscopy. The cleaned specimen is drawn out of the vacuum chamber into the second
chamber by a long rod and positioned under the microscope tip; here it can be imaged in
vacuum first, if desired.
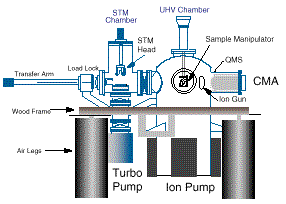 A CLEAN SINGLE-CRYSTAL METAL SAMPLE IS PREPARED IN THE HIGH-VACUUM CHAMBER
ON THE RIGHT, THEN TRANSFERRED TO THE CHAMBER CONTAINING THE SCANNING TUNNELING
MICROSCOPE, WHICH CAN BE PRESSURIZED AND HEATED.
|
Then, after a gate valve is closed between the chambers, one or more gases can be
introduced. In addition to carbon monoxide with platinum, Salmeron and his colleagues have
studied a mixture of carbon monoxide and molecular oxygen. Although pressures used to date
have been one atmosphere or less, pressures of hundreds of atmospheres are possible.
Samples are heated -- up to 500 degrees Kelvin, if desired -- using an ordinary
movie-projector lamp whose hot filament, sealed inside a glass bulb, cannot react with the
gas.
After working with platinum, carbon monoxide, and oxygen, Salmeron and his colleagues
have investigated the action of the gases with another important catalyst, rhodium. Under
heat and pressure, patterns never seen before appear, quite different from the hexagonal
moiré patterns evident on platinum. Studies like these may lead to a fundamental
understanding of catalytic processes and an ability to control and improve them.
"There is so much chemistry to be investigated at the atomic level," says
Salmeron, "that much of what we are doing is simply establishing a technique for
working under realistic conditions. We hope researchers will get enthusiastic about using
it on many other problems."
"High pressure adsorbate structures studied by scanning tunneling microscopy: CO
on Pt(111) in equilibrium with the gas phase," by Jensen et al, appeared in the 9
February 1998 Physical Review Letters. Work by Salmeron and his colleagues on
rhodium is in press. |